Introduction
Chronic kidney disease is an increasingly common health problem that is often undetected
Chronic kidney disease (CKD) is a major global public health problem. Based on data from the Ausdiab study (1), it is estimated that one in every 7 adults will have CKD, including one in 10 individuals with at least moderate kidney failure (defined as a glomerular filtration rate ŠGFRĆ < 60 mL/min/1.73m2). Similar findings have also been reported in North America (2) and Europe (3). Moreover, 1% of adults each year will develop new-onset CKD. Over the last 25 years, while the world’s population has grown by approximately 1.5% per annum, the number of individuals being treated with dialysis or kidney transplantation has increased more than 8% per annum (4). CKD is often not associated with significant symptoms and is unrecognized in 80-90% of cases (1,5,6). Its presence is a very strong risk factor for cardiovascular disease, such that individuals with CKD have up to a 10- to 20-fold greater risk of cardiac death than age- and sex-matched controls without CKD (7,8). For example, a 25 year old woman with advanced CKD has approximately the same risk of cardiovascular death as a 75 year old male without kidney disease (7). Furthermore, patients with CKD are at least 20 times more likely to die from cardiovascular disease than survive to the point of needing dialysis or kidney transplantation. Early identification and management of CKD is highly cost-effective and can reduce the risk of kidney failure progression and cardiovascular disease by 20% - 50% (9).
Recently, automated laboratory reporting of estimated GFR (eGFR) has been suggested as a simple, effective strategy for enhancing detection of CKD, thereby facilitating the timely institution of therapies proven to slow or prevent kidney failure progression, enhancing the appropriate assessment and modification of cardiovascular risk, and informing decisions regarding the prescription of drugs excreted by the kidneys (10). The aims of this article are to review the strengths, weaknesses and available evidence for the role of automated laboratory reporting of eGFR in CKD detection.
Assessment of kidney function: Role of estimated glomerular filtration rate
The most commonly used measure of overall kidney function in clinical practice is serum creatinine concentration. Unfortunately, this measurement is affected by many factors other than the level of kidney function and varies markedly with age, gender and muscle mass. Moreover, there are significant calibration issues associated with the measurement of serum creatinine that lead to inter-laboratory variation of up to 20% (11). Serum creatinine concentration is notoriously insensitive for detecting mild-to-moderate kidney failure, such that patients must lose 50% or more of their kidney function before the serum creatinine value rises above the upper limit of normal (12). This situation of a “normal” creatinine masking a significant decline in kidney function is especially important in elderly patients, in whom the age-related decline in kidney function is not reflected by an increase in serum creatinine level because of a concomitant decrease in muscle mass.
A number of low molecular weight serum proteins, including 2-microglobulin, retinol-binding protein and cystatin C, have been proposed as suitable alternative endogenous filtration markers (13-15). Of these, cystatin C has received the most interest in the published literature and appears to be superior to serum creatinine for detecting reduced kidney function, particularly in the early stages (16). However, the advantage of serum cystatin C compared with serum creatinine for the detection of kidney failure is no longer apparent when measurements are adjusted or stratified for age, gender and weight. Moreover, serum cystatin concentrations are significantly influenced by a number of factors other than GFR, including age, gender, body size, current cigarette smoking, serum C-reactive protein levels, corticosteroid treatment, cyclosporine A, thyroid dysfunction, physical activity, certain malignancies and pregnancy. There are also significant concerns pertaining to the cost-effectiveness of serum cystatin C for CKD detection.
Measuring GFR is widely accepted as the best overall index of kidney function (17,18). The most common method for assessing GFR in the past was performing a timed urine collection for evaluation of creatinine clearance. However, this test was inconvenient and frequently inaccurate as a result of improper collection and overestimation of GFR due to kidney tubular secretion of creatinine (18). Indeed, creatinine clearance measurements have generally been shown to provide less reliable estimates of GFR than GFR prediction equations (19). Commonly used exogenous filtration markers (iothalamate, DTPA, EDTA, iohexol) provide acceptable alternative measures of glomerular filtration rate (GFR), although their cost and labour-intensiveness limit their clinical utility as a screening tool for CKD.
More recently, calculation of estimated GFR (eGFR) using an empirical mathematical formula has been encouraged as a simple, rapid and reliable means of assessing kidney function (6,20-22). In most cases, eGFR is at least as accurate as measuring creatinine clearance (17). There are no fewer than 47 different prediction equations currently available, although the 2 most common in use are the Cockcroft-Gault (23) and the abbreviated Modification of Diet in Renal Disease (MDRD) formulae (20) (Table 1).
Table 1. Equations for estimated glomerular filtration rate (eGFR) in adults based on serum creatinine concentration*.
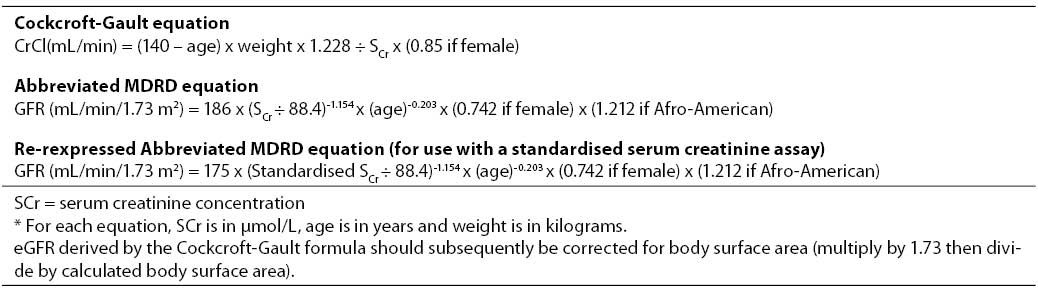
The Cockcroft-Gault formula was originally derived in 249 consecutive hospitalised patients (96% male, age range 18–92 years) at the Queen Mary Veterans’ Hospital in Canada, based on the means of two 24-hour creatinine clearances (23). Serum creatinine concentrations were determined by Jaffé reaction using an autoanalyzer (N-11B, Technicon Instruments Corp, NY). The derived formula was then used to predict creatinine clearance in a second validation cohort consisting of 236 patients (206 males, mean creatinine clearance 72.7 36.6 mL/min). The Cockcroft-Gault equation has the advantages of being more widely known, easier to remember and more extensively validated than the MDRD formula. Although the equation was developed in hospitalised, white men, many of whom did not have CKD, it has subsequently been extensively validated and found to exhibit satisfactory precision and bias in diverse populations including women and various ethnic groups, and across a broad range of GFRs. The principal disadvantages of the Cockcroft-Gault formula are the requirement to measure weight and height (the latter is required for the purposes of body surface area correction), its estimation of creatinine clearance rather than GFR, and the inability of clinical laboratory creatinine assays to be calibrated to the laboratory that performed the assays on samples used to derive the Cockcroft-Gault equation.
In contrast, the MDRD equation was developed in 1628 CKD patients enrolled in the baseline period of the Modification of Diet in Renal Disease (MDRD) study, of whom 1070 were randomly selected as the derivation sample and the remaining 558 patients constituted the validation sample (20). Using multiple regression analysis, a 6-variable equation was initially developed, which included the variables of serum creatinine, age, gender, ethnicity (African-American or other), serum urea and serum albumin. The equation was validated against GFR corrected for body surface area (BSA) and so, unlike the Cockcroft-Gault formula, the predicted GFR is expressed as mL/min/1.73 m2 and does not require subsequent BSA normalisation. Subsequently, a comparably accurate 4-variable MDRD equation consisting of serum creatinine, age, gender and ethnicity was proposed to simplify clinical use (24). The equation was re-expressed in 2005 for use with a standardised serum creatinine assay, which yields 5% lower values for serum creatinine concentration (25). The advantages of the MDRD formula are that it does not require knowledge of the patient’s weight (making it far more suitable for automated laboratory reporting), does not need correction for body surface area (and therefore does not require knowledge of the patient’s height) and has been generally shown to be more precise and accurate than the Cockcroft-Gault equation when the GFR is below 60 mL/min/1.73 m2 (validated in over 10,000 subjects) (reviewed in (22)). Both the MDRD and Cockcroft-Gault prediction formulae perform poorly in patients with normal or near-normal renal function, including healthy kidney donors (26-28) and early diabetics (29-30).
International guidelines for automated laboratory reporting of eGFR
In North America, the National Kidney Foundation Kidney and Dialysis Outcomes Quality Initiative (K/DOQI) guidelines have recommended that serum creatinine concentration alone is sub-optimal for assessing the level of kidney function and that pathology laboratories should concomitantly report eGFR, as determined by a prediction equation, such as the MDRD formula (17). Similar recommendations have been made by other organizations, including the British Renal Association (31) and the Kidney Disease Improving Global Outcomes (KDIGO) guidelines (32). These recommendations are based on observations that laboratory reporting of eGFR significantly enhances CKD detection (21) and that timely intervention can reduce the risk of progressive kidney failure and cardiovascular disease in CKD patients (9).
In August 2005, an Australasian eGFR Working Group convened by representatives of the Australasian Association of Clinical Biochemists (AACB), Australian and New Zealand Society of Nephrology (ANZSN), Kidney Health Australia (KHA) and Royal Australasian College of Pathologists (RCPA) unanimously endorsed and published recommendations that laboratories calculate and report an eGFR using the MDRD formula with every request for serum creatinine concentration (33):
- An eGFR using the abbreviated MDRD formula shall be automatically calculated for every request for serum creatinine concentration in individuals aged ≥ 18 years.
- GFR values that calculate to be in excess of 60 mL/min/1.73m2 should be reported as “>60mL/min/1.73m2” and not as a precise figure.
- Automatic reporting of eGFR may include age-related reference intervals for individuals aged ≥ 65 years.
- The implementation of automatic eGFR reporting will require a timely educational program that ensures information is available to health professionals to aid in interpretation of eGFR values.
All Australian and New Zealand pathology laboratories now automatically report eGFR in any adult with a request for serum creatinine determination.
Importance of standardisation of creatinine assays
Lack of calibration of serum creatinine assays can represent a source of significant error in the estimation of GFR by empiric formulae. Calibration of serum creatinine measurements is not standardised, thereby leading to substantial variation within and between laboratories. Of 11 common biochemical tests evaluated across 700 laboratories by the College of American Pathologists, differences in calibration of serum creatinine assays (approximately 18 mol/L between labs) accounted for 85% of the difference between laboratories and were greater than for any of the other 10 analytes examined (34). Serum creatinine concentration was over-estimated by an average of 13.3% compared with a reference method. Calibration biases appear to be particularly important at lower serum creatinine levels. Similar results were found among the 102 laboratories participating in the Nordic Reference Interval Project (NORIP) (35). A more recent evaluation of participants in the College of American Pathologists Chemistry Survey demonstrated that the mean bias for 50 instrument-method peer groups varied from -0.06 to 0.31 mg/dL (-5.3 to 27.4 µmol/L), with 30 (60%) of 50 peer groups having significant bias (P < 0.001) (36). The bias variability was related to instrument manufacturer rather than method type, with 24 (63%) of 38 alkaline picric acid methods and with 6 (50%) of 12 enzymatic methods having significant biases.
Murthy et al (37) recently computed errors in GFR estimates based on the range of calibration differences from the 1994 College of American Pathologists survey using MDRD eGFR. Not surprisingly, errors were higher at higher level of eGFR (and lower levels of serum creatinine) and became clinically important at an eGFR value of 60 mL/min/1.73 m2, at which point the 95% confidence interval for the mean calibration difference was associated with a maximal range of error in GFR estimates from +4.6 to -18.1 mL/min/1.73 m2 (+7.6 to -30.2%). Based on these results, the K/DOQI (32) and Australasian (33) guidelines both recommend that clinical laboratories report a specific value for GFR estimates only when the estimated GFR is less than 60 mL/min/1.73m2 and as “60 mL/min/1.73m2” for higher values. The National Kidney Disease Education Program (NKDEP) has launched the Creatinine Standardization Program to address inter-laboratory variation in creatinine assay calibration and provide more accurate estimates of GFR. A similar process has already taken place in Australasia, such that most creatinine assays are generally aligned with isotope-dilution mass spectrometry (IDMS). Consequently, the Australasian eGFR Working Group has recommended using the re-expressed (“175”) MDRD formula for automated laboratory reporting. The International Federation of Clinical Chemistry and Laboratory Medicine Working Group on Standardisation of Glomerular Filtration Rate Assessment (IFCC:WG-GFRA) is currently considering proposals to establish a reference laboratory network for creatinine to assist manufacturers in validating traceability of their methods and to introduce guidelines for global introduction of eGFR applying the revised abbreviated MDRD equation.
Limitations of automated eGFR reports
There are a number of limitations associated with eGFR:
- rapidly changing kidney function (eg acute kidney failure);
- dialysis-dependent patients;
- exceptional dietary intake (eg vegetarian diet, high protein diet, creatine supplements);
- extremes of body size;
- diseases of skeletal muscle, paraplegia, those with high muscle mass and amputees;
- severe liver disease;
- children (under 18 years);
- eGFR values above 60 mL/min/1.73 m2;
- Aboriginal and Torres Strait Islander peoples;
- Asian populations (including Japanese, Chinese and Vietnamese);
- Maori and Pacific Islander peoples.
These limitations include errors related to serum creatinine measurement, such as random errors, short-term variations in renal function, variations in tubular creatinine secretion, variations in extra-renal (gut) creatinine excretion and variations in creatinine generation by muscle or dietary intake. Moreover, prediction formulae for eGFR have been poorly validated in children (38) and so their use is currently only recommended in adults ( 18 years). After the age of 30 years, GFR progressively declines at an average rate of 8 mL/min per decade (39). Based on North American data (39), it is estimated that 25% of the population over the age of 70 years will have an eGFR below 60 mL/min/1.73 m2. While most studies show that GFR declines with age, accepting this as normal runs the risk of “normalising” a pathological state caused by age-related diseases rather than age itself. A fall in GFR is not an inevitable consequence of ageing with the Baltimore Longitudinal Study on Ageing showing that the decline in GFR with age was largely accountable for by hypertension (40). Moreover, a reduced GFR remains a strong predictor of all-cause and cardiovascular mortality, even in elderly populations (41-43). Indeed, the impact of reduced GFR appears largely independent of age, although one large mortality study has demonstrated a weaker association of mortality in the elderly than in the younger groups (44). The Australasian eGFR Working Group has suggested that automatic laboratory reporting of eGFR may include age-related reference intervals for individuals aged 65 years. However, the definition of CKD is not modified according to age. This scenario is analogous to that for hypertension. Even though blood pressure levels rise with age and are highly prevalent in the elderly in the developed world, the threshold for diagnosing hypertension based on blood pressure level is not altered in older individuals because hypertension in this age group is still strongly associated with adverse outcomes. Similarly, severely reduced eGFR values in elderly patients below 60 mL/min/1.73 m2 should be considered significant. The Australasian eGFR Working Group recently revisited this issue in December 2006 and concluded that at this time it was premature to recommend age-related decision points for eGFR but that it was appropriate to advise practitioners that, in those of 70 years and older, an eGFR from 45 to 59 mL/min/1.73m2, when stable over time and unaccompanied by other evidence of kidney damage, may be interpreted as consistent with a typical eGFR for this age and unlikely to be associated with CKD complications. Importantly, this commonly found moderate reduction in eGFR in the elderly should always be considered when drug dosing decisions are being made about renally excreted drugs.
In addition to extremes of age, there are a number of other clinical situations where reported eGFR results should be interpreted with caution due either to lack of appropriate validation or to demonstrated lack of precision or accuracy (eg with eGFR values above 60 mL/min/1.73 m2). The CARI Guidelines (19) currently recommend that direct measurement of GFR (e.g. by creatinine clearance or one of the GFR reference methods) may be required in situations in which eGFR may be unreliable or in which a high degree of accuracy in GFR estimation is required (such as prior dosing with renally-excreted medications that have high toxicity or evaluation of renal function in potential live kidney donors).
Interpretation of eGFR values on pathology reports
The normal GFR in young adults is around 120 mL/min/1.73 m2. eGFR values below 60 mL/min/1.73 m2 are abnormal and generally indicate the presence of CKD if present for more than 3 months. In individuals with an eGFR value > 60 mL/min/1.73 m2, CKD considered to be present if there is concomitant evidence of kidney damage (including microalbuminuria, macroalbuminuria, persistent glomerular haematuria or radiological renal abnormalities). The K/DOQI and CARI guidelines have classified five different stages of CKD based on the level of GFR (17,45). These are listed in Table 2, together with a clinical action plan based on eGFR level.
Table 2. Classification of CKD stage
Patients with a significant reduction in eGFR (<60 mL/min/1.73 m2) are at significantly increased risk of cardiovascular disease and renal failure progression. At a minimum, such patients should be investigated with an urinary protein:creatinine ratio, blood pressure measurement and renal ultrasongraphy. The principal goals of CKD management are:
a) reduction of cardiovascular and renal risk (lifestyle modification, antiproteinuric agents, blood pressure control, cholesterol control, glycaemic control, weight reduction, smoking cessation, partial anaemia correction);
b) early detection and management of CKD complications (such as hypertension, secondary hyperparathyroidism, renal osteodystrophy, anaemia, sleep apnoea, restless legs, cardiovascular disease, and malnutrition);
c) avoidance of nephrotoxic medications and evaluation of prescribed medications to ensure that dosages are appropriate for the level of kidney function; and,
d) timely referral of CKD patients to a nephrologist in the limited circumstances where this is appropriate (Table 3 lists the current indications for nephrologist referral, as recommended by the Kidney Check Australia Taskforce).
Table 3. Indications for referral of CKD patients to a nephrologist.
Use of eGFR for drug dosing
The appropriate prescribing of many drugs depends on knowledge of the patient’s renal function. There is wide agreement that decision making in drug dose adjustment in people with CKD is enhanced by an assessment of kidney function based on GFR rather than a serum creatinine concentration alone. Although the MDRD formula provides a more reliable estimate of GFR than that of Cockcroft-Gault, the latter is currently considered optimal for drug dosing because most of the renal dosing recommendations are based on Cockcroft-Gault creatinine clearance and because drug dosing should be based on actual GFR (mL/min) rather than GFR normalised to body surface area (mL/min/1.73 m2) (46). On the other hand, there are concerns about the variability in the recommended use of the C-G formula with regard to use of estimated ideal or actual body weight and the use of an outdated formula which has not been revised to account for changes in serum creatinine assay re-standardisation. Use of both the Cockcroft-Gault and MDRD equations mostly results in concordant prescribing recommendations, but a recent study suggested that potentially clinically important differences in clinical decision-making occurred in 21-37% of patients (47). It is not yet known whether the use of the Cockcroft-Gault or the MDRD eGFR for drug dosing results in superior clinical outcomes. Most guideline groups cautiously recommend using the Cockcroft-Gault creatinine clearance for drug dosing until more clinical studies with the MDRD formula are performed, although the Australasian eGFR Working Party recommends that it appears reasonable and indeed preferable, in the absence of any other measure of kidney function, to use the MDRD eGFR (recognising its limitations) as a guide to prescribing particularly with non-critical dose drugs in general practice. At the very least, the MDRD eGFR alerts treating doctors to the possibility of reduced renal function to allow the use of other estimates, if desired.
The impact of automatic laboratory eGFR reporting on CKD detection
Automatic eGFR reporting has been shown to result in enhanced detection of CKD in the community and improved referral of CKD patients to nephrologists. Akbari et al (2004) conducted a before-and-after study of 324 patients aged ≥ 65 years at an outpatient family medicine practice. The intervention consisted of automatic laboratory reporting of Cockcroft-Gault creatinine clearance together with an educational intervention directed at primary care physicians. Recognition of CKD (GFR < 60 mL/min) by the primary care physician was the primary outcome measure and was significantly increased by the intervention increasing from 22.4% to 85.1% of patients with CKD. Increased recognition of CKD leads to increased referral rates of mostly appropriate patients, important in concentrating limited resources. An audit in 2006 in Australia looked at referrals to a tertiary hospital, a provincial hospital and a private practice pre and post introduction of automated eGFR reporting. Overall the referral rates only increased in the tertiary hospital by approximately 53% and the quality, as adjudicated by the KCAT guidelines for referral, remained unchanged with two thirds appropriately referred.
It remains to be determined whether routine reporting of eGFR will improve CKD outcomes in the community, although a randomised controlled trial is currently underway in Canada. Nevertheless, in a non-randomised controlled trial of 52 diabetic patients in whom CKD was detected in primary practice on the basis of an abnormal eGFR report and/or proteinuria, Martinez-Ramirez et al (48) demonstrated that patients who were subsequently referred to a nephrologist exhibited better preservation of renal function than those who remained treated by only their family doctors. Other studies have also suggested that early detection of CKD and timely intervention with renoprotective therapies is associated with a 20%-50% reduction in cardiovascular and renal risk (9).
Conclusions
Automatic laboratory reporting of eGFR on each occasion a serum creatinine concentration is ordered will significantly increase the likelihood of early detection of CKD, allow the institution of appropriate management strategies to reduce the risks of kidney failure progression and cardiovascular death in the community, and inform decisions regarding the prescription of renally excreted medications. Clinical chemists need to be aware of the need to achieve better standardisation of serum creatinine measurements and traceability of all measurements to enhance the diagnostic accuracy of automated eGFR reports (especially at lower serum creatinine values).
References
1. Chadban SJ, Briganti EM, Kerr PG, Dunstan DW, Welborn TA, Zimmet PZ et al. Prevalence of kidney damage in Australian adults: The AusDiab kidney study. J Am Soc Nephrol 2003;14: S131-8.
2. Clase CM, Garg AX, Kiberd BA. Prevalence of low glomerular filtration rate in nondiabetic Americans: Third National Health and Nutrition Examination Survey (NHANES III). J Am Soc Nephrol. 2002;13:1338-49.
3. Hallan S, Astor B, Lydersen S. Estimating glomerular filtration rate in the general population: the second Health Survey of Nord-Trondelag (HUNT II). Nephrol Dial Transplant 2006;21:1525-33.
4. Moeller S, Gioberge S, Brown G. ESRD patients in 2001: global overview of patients, treatment modalities and development trends. Nephrol Dial Transplant 2002;17: 2071-6.
5. McClellan WM, Knight DF, Karp H, Brown WW. Early detection and treatment of renal disease in hospitalized diabetic and hypertensive patients: important differences between practice and published guidelines. Am J Kidney Dis 1997;29:368-75.
6. John R, Webb M, Young A, Stevens PE. Unreferred chronic kidney disease: a longitudinal study. Am J Kidney Dis 2004;43:825-35.
7. Foley RN, Parfrey PS, Sarnak MJ. Clinical epidemiology of cardiovascular disease in chronic renal disease. Am J Kidney Dis 1998;32:S112-9.
8. Weiner DE, Tighiouart H, Amin MG, Stark PC, MacLeod B, Griffith JL et al. Chronic kidney disease as a risk factor for cardiovascular disease and all-cause mortality: a pooled analysis of community-based studies. J Am Soc Nephrol 2004;15: 1307-15.
9. Johnson DW. Evidence-based guide to slowing the progression of early renal insufficiency. Intern Med J 2004;34:50-7.
10. Johnson DW, Usherwood T. Automated reporting of glomerular filtration rate: coming soon to a laboratory near you! Aust Fam Physician 2005;34:925-31.
11. Coresh J, Astor BC, McQuillan G, Kusek J, Greene T, Van Lente F et al. Calibration and random variation of the serum creatinine assay as critical elements of using equations to estimate glomerular filtration rate. Am J Kidney Dis 2002;39:920-9.
12. Johnson DW. Use of serum creatinine concentration to assess level of kidney function. Nephrology 2005;10:S133-9.
13. Grubb A, Simonsen O, Sturfelt G, Truedsson L, Thysell H. Serum concentration of cystatin C, factor D and beta 2-microglobulin as a measure of glomerular filtration rate. Acta Med Scand 1985;218:499-503.
14. Donadio C, Lucchesi A, Ardini M, Giordani R. Cystatin C, beta 2-microglobulin, and retinol-binding protein as indicators of glomerular filtration rate: comparison with plasma creatinine. J Pharm Biomed Anal 2001;24:835-42.
15. Filler G, Witt I, Priem F, Ehrich JH, Jung K. Are cystatin C and beta 2-microglobulin better markers than serum creatinine for prediction of a normal glomerular filtration rate in pediatric subjects? Clin Chem 1997;43:1077-8.
16. Johnson DW. Use of cystatin C measurement in evaluating kidney function. Nephrology 2005;10:S157-67.
17. K/DOQI clinical practice guidelines for chronic kidney disease: evaluation, classification, and stratification. Am J Kidney Dis 2002;39:S1-266.
18. Johnson CA, Levey AS, Coresh J, Levin A, Lau J, Eknoyan G. Clinical practice guidelines for chronic kidney disease in adults: Part II. Glomerular filtration rate, proteinuria, and other markers. Am Fam Physician 2004;70:1091-7.
19. Johnson DW. Direct measurement of glomerular filtration rate. Nephrology 2005;10: S168-71.
20. Levey AS, Bosch JP, Lewis JB, Greene T, Rogers N, Roth D. A more accurate method to estimate glomerular filtration rate from serum creatinine: a new prediction equation. Modification of Diet in Renal Disease Study Group. Ann Intern Med 1999; 130:461-70.
21. Akbari A, Swedko PJ, Clark HD, Hogg W, Lemelin J, Magner P et al. Detection of chronic kidney disease with laboratory reporting of estimated glomerular filtration rate and an educational program. Arch.Intern Med 2004;164:1788-92.
22. Johnson DW. Use of estimated glomerular filtration rate to assess level of kidney function. Nephrology 2005;10:S140-6.
23. Cockcroft DW, Gault MH. Prediction of creatinine clearance from serum creatinine. Nephron 1976;16:31-41.
24. Levey AS, Greene T, Kusek JW, Beck GJ. A simplified equation to predict glomerular filtration rate from serum creatinine. J Am Soc Nephrol 2000;11;AO828 (Abstract).
25. Levey AS, Coresh J, Greene T, Stevens LA, Zhang YL, Hendriksen S et al. Using standardized serum creatinine values in the modification of diet in renal disease study equation for estimating glomerular filtration rate. Ann Intern.Med 2006;145:247-54.
26. Poggio ED, Wang X, Greene T, Van Lente F, Hall PM. Performance of the Modification of Diet in Renal Disease and Cockcroft-Gault equations in the estimation of GFR in health and in chronic kidney disease. J Am Soc Nephrol 2005; 16:459-66.
27. Rule AD, Gussak HM, Pond GR, Bergstralh EJ, Stegall MD, Cosio FG, et al. Measured and estimated GFR in healthy potential kidney donors. Am J Kidney Dis 2004;43:112-9.
28. Lin J, Knight EL, Hogan ML, Singh AK. A comparison of prediction equations for estimating glomerular filtration rate in adults without kidney disease. J Am Soc Nephrol 2003;14:2573-80.
29. Chudleigh RA, Dunseath G, Evans W, Harvey JN, Evans P, Ollerton R, et al. How reliable is estimation of glomerular filtration rate at diagnosis of type 2 diabetes? Diabetes Care 2007;30:300-5.
30. Fontsere N, Salinas I, Bonal J, Bayes B, Riba J, Torres F, et al. Are prediction equations for glomerular filtration rate useful for the long-term monitoring of type 2 diabetic patients? Nephrol Dial Transplant 2006;21:2152-8.
31. Joint Specialty Committee of the Royal College of Physicians of London and the British Renal Association. Guidelines for identification, management and referral of adults with chronic kidney disease. Department of Health for England, London, 2005; 20-3.
32. Eknoyan G, Lameire N, Barsoum R, Eckardt KU, Levin A, Levin N, et al. The burden of kidney disease: improving global outcomes. Kidney Int 2004;66:1310-4.
33. The Australasian eGFR Working Party. Chronic kidney disease and automatic reporting of estimated glomerular filtration rate: a position statement. Med J Aust 2005;183:138-41.
34. Ross JW, Miller WG, Myers GL, Praestgaard J. The accuracy of laboratory measurements in clinical chemistry: a study of 11 routine chemistry analytes in the College of American Pathologists Chemistry Survey with fresh frozen serum, definitive methods, and reference methods. Arch Pathol Lab Med 1998;122:587-608.
35. Rustad P. Reference intervals for 25 of the most frequently used properties in clinical chemistry: Proposal by the NORDIC Reference Interval Project (NORIP). Klinisk kemi Norden 2003;15:10-7.
36. Miller WG, Myers GL, Ashwood ER, Killeen AA, Wang E, Thienpont LM, et al. Creatinine measurement: state of the art in accuracy and interlaboratory harmonization. Arch Pathol Lab Med 2005;129:297-304.
37. Murthy K, Stevens LA, Stark PC, Levey AS. Variation in the serum creatinine assay calibration: a practical application to glomerular filtration rate estimation. Kidney Int 2005;68:1884-7.
38. Pierrat A, Gravier E, Saunders C, Caira MV, Ait-Djafer Z, Legras B, et al. Predicting GFR in children and adults: a comparison of the Cockcroft-Gault, Schwartz, and modification of diet in renal disease formulas. Kidney Int 2003;64:1425-36.
39. Coresh J, Astor BC, Greene T, Eknoyan G, Levey AS. Prevalence of chronic kidney disease and decreased kidney function in the adult US population: Third National Health and Nutrition Examination Survey. Am J Kidney Dis 2003;41:1-12.
40. Lindeman RD, Tobin J, Shock NW. Longitudinal studies on the rate of decline in renal function with age. J Am Geriatr Soc 1985;33:278-85.
41. Fried LP, Kronmal RA, Newman AB, Bild DE, Mittelmark MB, Polak JF, et al. Risk factors for 5-year mortality in older adults: the Cardiovascular Health Study. JAMA 1998;279:585-92.
42. Shlipak MG, Heidenreich PA, Noguchi H, Chertow GM, Browner WS, McClellan MB. Association of renal insufficiency with treatment and outcomes after myocardial infarction in elderly patients. Ann Intern Med 2002;137:555-62.
43. Manjunath G, Tighiouart H, Coresh J, MacLeod B, Salem DN, Griffith JL, et al. Level of kidney function as a risk factor for cardiovascular outcomes in the elderly. Kidney Int 2003;63:1121-9.
44. O’Hare AM, Bertenthal D, Covinsky KE, Landefeld CS, Sen S, Mehta K, et al. Mortality risk stratification in chronic kidney disease: one size for all ages? J Am Soc Nephrol 2006;17:846-53.
45. Johnson DW. Classification of chronic kidney disease based on evaluation of kidney function. Nephrology 2005;10:S172-6.
46. Faull R. Prescribing in renal disease. Aust Prescr 2007;30:17-20.
47. Wargo KA, Eiland EH, III, Hamm W, English TM, Phillippe HM. Comparison of the modification of diet in renal disease and Cockcroft-Gault equations for antimicrobial dosage adjustments. Ann Pharmacother 2006;40:1248-53.
48. Martinez-Ramirez HR, Jalomo-Martinez B, Cortes-Sanabria L, Rojas-Campos E, Barragan G, Alfaro G, et al. Renal function preservation in type 2 diabetes mellitus patients with early nephropathy: a comparative prospective cohort study between primary health care doctors and a nephrologist. Am J Kidney Dis 2006;47:78-87.