Introduction
The effect of morphine-like psychoactive drugs has long been a subject of scientific interest, especially considering their therapeutic effects in the treatment of psychiatric disorders, anxiety, depression, etc. The research into the relation between endorphins and depression began with the findings of encephalin and opioid receptors located in the mood-response areas of the brain. Previous studies have raised a question whether excess, deficient, or static levels of endorphins cause depression. Endorphins are likely to modulate the nervous system activity over a long-term rather than short-term period.
Endorphins are released in the conditions of shock, freeze, ”fight or flight”, trauma, physical pain, and in all stress situations including psychological stress. They serve as an analgesic, anesthetic and cause dissociation, immobilization and loss of self. Depressors induce elevation of stress hormone levels in blood. Since endorphins are released along with ACTH in response to any stressor, depressors are supposed to “elevate” endorphin levels as well.
Research in the field of psychopharmaceuticals has provided considerable knowledge about their favorable effects as well as side effects. Tricyclic antidepressants were introduced first, showing effects as a supplement for the primary role of inhibition of norepinephrine and serotonin uptake by the nervous ends (1,2).
Although the efficacy of selective serotonin reuptake inhibitors (SSRIs) and tricyclic antidepressants is similar, the SSRIs have better safety and tolerability profile, making them preferable agents for all anxiety disorders. The considerable side effect burden, the potential of producing anticholinergic delirium, and their contribution to the occurrence of falls and hip fractures make tricyclic antidepressants especially unsuitable for the elderly and are therefore no longer recommended (3).
Trazodone is an effective antidepressant with a broad therapeutic spectrum, including anxiolytic efficacy. Because of its sedative effect, this triazolopyridine antidepressant is currently the second most commonly prescribed agent for the treatment of insomnia. Considering the widespread use of trazodone, careful review of the literature was performed to assess its efficacy and side effects when used for the treatment of insomnia. Although trazodone is usually referred to as a serotonin (5-HT) reuptake inhibitor, this pharmacological effect appears to be too weak to fully account for its clinical effectiveness (4,5).
The aim of our study was to establish the possible influence of psychotropic drugs on serum and brain β-endorphins in rats as experimental model.
Materials and methods
Animals
Thirty male albino Wistar rats (200–250 g body weight) were divided into groups of 6 animals. Trazodone (5 mg/kg/day) was administered to the experimental group, and 0.95% NaCl solution to the control group. Blood samples, about 0.5 mL per day of treatment, were collected from the great tail vein. After 30 minutes at room temperature, serum was obtained by centrifugation at 3000 rpm for 10 minutes, separated from clot and frozen at -20 °C until analysis. Quantification of serum β-endorphins was performed before (day 0), and on days 1, 9 and 28 of trazodone administration, whereby each animal served as its own control.
To establish the extent of psychotropic influence, we performed an additional study of brain β-endorphins. Prior to the collection of brain samples, all animals were properly sacrificed. Collection of whole brain was performed immediately in control group, and on days 1, 9 and 28 of trazodone administration in experimental group. After homogenization in a Potter’s homogenizer, tissue samples were centrifuged at 8000 rpm for 20 minutes. Clear supernatants were placed in tubes and frozen at -20 °C until analysis.
The experiment was approved by the Ethics Committee of the School of Medicine, University of Sarajevo.
Methods
The radioimmunoassay (RIA) kit (Nichols Institute, San Juan, Capistrano, USA) was employed for quantification of serum and brain β-endorphins (6). For radioactivity level measurement, β-counter with a gamma-radiation source (LKB Wallac, Sweden) was used. The concentrations of β-endorphins are directly proportional to the radioactivity measured in the samples. Concentrations are given in pg/mL for sera, and in pg/g of brain material for brain β-endorphin concentrations.
Statistical analysis
Statistical evaluation of serum endorphin concentrations was performed using the SigmaStat 2.03 (SPSS Inc. 1995) software. Repeatedly measured values were analyzed using Friedman RM ANOVA to determine major effects on serum β-endorphin concentrations over time after drug application. Tukey’s post hoc test for multiple comparisons was used to determine significant differences in serum β-endorphin levels on particular days of drug application (day 0 – day 28).
On statistical evaluation of brain β-endorphin concentration between the groups, we used Kruskal-Wallis one-way ANOVA. Bonferroni t-test for multiple comparisons was used to determine the significance of differences in the brain β-endorphin concentration. All data are expressed as mean, standard deviation (SD), and standard error of mean (SEM). The level of significance was set at P < 0.05.
Results
Results of serum and brain concentrations of β-endorphins measured on particular days of trazodone application are given in Table 1. Serum concentrations measured on day 1 of trazodone application were significantly higher (72.31 ± 1.86 pg/mL; x ± SEM) compared to initial values (45.83 ± 3.77 pg/mL; P = 0.001). However, significantly lower β-endorphin concentrations were recorded on day 9 (33.4 ± 1.91 pg/mL) compared to day 1 of treatment (P = 0.001). These values were also significantly lower than the values measured on day 0 (P = 0.011). The last determination of serum β-endorphins was done on day 28 of trazodone application. These values (38.62 ± 1.42 pg/mL) were lower than the initial values (P = 0.189), and significantly lower than the values recorded on day 1 of treatment (P = 0.001). The values obtained on day 28 were higher compared to the values on day 9, however, without statistical significance (P = 0.439).
Table 1. Serum and brain concentrations of β-endorphins according to days of trazodone treatment (mean ± standard deviation)
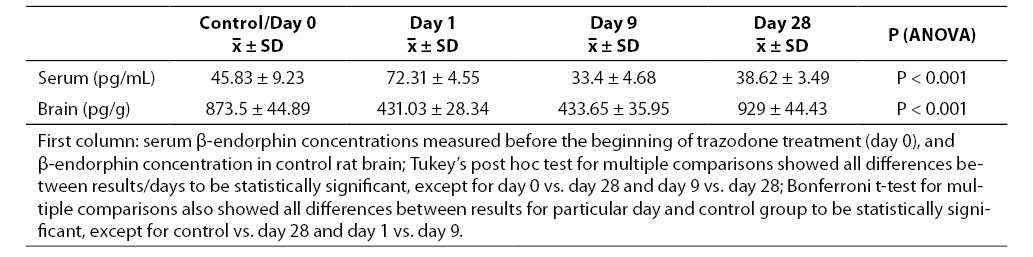
Data on the rat brain β-endorphin concentration showed a significant decrease on day 1 of trazodone administration (431.03 ± 11.57 pg/g) compared to control rat brains (873.5 ± 18.32 pg/g; P = 0.001). A statistically significant difference vs. control group was also recorded on day 9 of treatment (433.65 ± 14.67 pg/g; P = 0.001). However, there was no statistically significant difference in the concentrations of brain β-endorphins recorded on day 1 and day 9 (P = 1.00). Finally, the brain β-endorphin concentrations measured on day 28 of trazodone application were significantly higher (929 ± 18.13 pg/g) in comparison with the concentrations recorded on either day 1 or day 9 of treatment (P = 0.001 both). The brain β-endorphin concentrations measured on day 28 of trazodone application were slightly higher than those recorded in control rat brains, however, the difference did not reach statistical significance (P = 0.137).
Discussion
Previous studies have demonstrated the synergistic influence of trazodone combined with mud bath in improving the psychological response to stress. Desipramine and paroxetine, used in animal depression models, did not significantly affect the extracellular levels of β-endorphins in nucleus accumbens. Chronic antidepressant treatment normalized serotonin-induced release of β-endorphins as well as behavioral manifestation of depression (7,8).
There still are relatively little data showing that the acute or chronic antidepressant treatment has different influence on serum and brain β-endorphin levels, as a potential factor in the mechanism of antidepressant action. In our study, serum β-endorphin concentrations showed a rapid and significant increase on day 1 of trazodone administration (72.31 ± 1.86 pg/mL), followed by a decrease on day 9 (33.4 ± 1.91 pg/mL) and reaching slightly lower values on day 28 of treatment (38.62 ± 1.42 pg/mL) as compared to initial values (45.8 ± 3.77 pg/mL). Data reported in the literature also point to changes in serum β-endorphin concentrations after chronic use of different antidepressants (9,10). Data obtained in the present study with trazodone are inconcordance with the report by Đurović et al. (9,10). The rapid increase in serum β-endorphin concentrations and changes observed on days 9 and 28 are indicative of both acute and chronic response to trazodone administration.
Previous studies have shown that acute amitriptyline and clomipramine produce naloxone-reversible antinociception. This apparent opioid-like involvement was further investigated by measuring β-endorphin levels in the hypothalamus following acute and chronic treatment with these antidepressants, demonstrating significantly raised levels of β-endorphins. The support was provided for the suggestion that antidepressants activate opioid systems through both direct opioid receptor interaction and an indirect action through the enhanced release of opioid peptides. Moreover, it has been postulated that the direct action of antidepressants on opioid receptors and the endogenous opioid peptides released interact as agonists at both μ- and δ-opioid receptors to inhibit nociceptive transmission, since the activity is antagonized by both naloxone and naltrindole (11).
Changes in serum β-endorphin levels should be followed by changes in their brain levels. In our study, a significant decrease of brain β-endorphin levels (431.03 ± 11.57 pg/g) was recorded on day 1, and was also observed in rat brains on day 9 of continuous antidepressant administration (433.65 ± 14.67 pg/g), as compared to control group values (873.50 ± 18.32 pg/g). On day 9, brain concentrations of β-endorphins were not different than the concentration measured on day 1 (Table 1).
In contrast, data obtained on day 28 of chronic treatment showed a significant increase in brain β-endorphins (929 ± 18.13 pg/g) compared to other days of trazodone treatment. Comparable results have been reported by Gray et al. (11), who used other antidepressants in their study. This suggests a possible increase in the brain β-endorphin synthesis in response to chronic trazodone administration.
Limitations of our study lie in the fact that serum β-endorphin concentrations were not recorded in animals used for quantification of brain β-endorphin concentration. However, we consider our results of brain β-endorphin concentration in trazodone treated animals relevant in comparison with the values obtained in control group.
The changes in serum and brain β-endorphins could be caused by differences in the acute or chronic triazolopyridine (trazodone) action. It points to possible differences in the rate of synthesis of β-endorphins, degradation of brain β-endorphins and their possible release to the blood stream, caused by continuous antidepressant administration.
Conclusion
Chronic trazodone treatment causes an increase in the brain synthesis of β-endorphins, while acute drug administration results only in a rapid release of β-endorphins into the circulation. We consider that serum and brain β-endorphin levels could be used for monitoring the psychoactive drug effects.
References
1. Brunton LL, Parker KL, Buxton ILO, Blumenthal DK. Gudman & Gilman’s The pharmacological basis of therapeutics, Eleventh edition, The McGraw-Hill Companies, New York, St. Louis, 2007.
2. Jadrić R, Zulić I, Hasić S, Kiseljaković E, Zečević B, Radovanović J, et al. Trazodone influence on rat sera beta-endorphins level. Bosn J Basic Med Sci 2004;4:33-6.
3. DeVane CL, Chiao E, Franklin M, Kruep EJ. Anxiety disorders in the 21st century: status, challenges, opportunities, and comorbidity with depression. Am J Man Care 2005;11:344-53.
4. Odagaki Y, Toyoshima R, Yamauchi T. Trazodone and its active metabolite m-chlorophenylpiperazine as partial agonists at 5-HT1A receptors assessed by (35S) GTP gammaS binding. J Psychopharmacol 2005;19:235-41.
5. Mendelson WB. A review of the evidence for the efficacy and safety of trazodone in insomnia. J Clin Psychiatry 2005;66:469-76.
6. Kaplan AL, Pesce AJ. Clinical chemistry – theory, analysis and correlation. Third edition, Mosby-Year Book, Inc., 1996.
7. Bellometi S, Galzigna L. Function of the hypothalamic adrenal axis in patients with fibromyalgia syndrome and undergoing mud-pack treatment. Int J Clin Pharmacol Res 1999;19:27-33.
8. Zangen A, Nakash R, Roth-Deri I, Overstreet DH, Yadid G.Impaired release of beta-endorphin in response to serotonin in a rat model of depression. Neuroscience 2002;110:389-93.
9. Đurović D, Milić-Aškrabić J, Majkić-Singh N. Effect o fluvoxamine on the level of beta-endorphin in the sera and nervous tissue of rats. Pharmazie 1998; 53:143-4.
10. Đurović D, Milić-Aškrabić J, Majkić-Singh N. Serum beta-endorphin level in patients with depression on fluvoxamine. Farmaco 1999; 54:130-3.
11. Gray AM, Spencer PSJ, Sewell RDE. The involvement of the opioidergic system in the antinociceptive mechanism of action of antidepressant compounds. Br J Pharmacol 1998;124: 669-74.