Introduction
Copeptin, a 39-aminoacid glycopeptide is a C-terminal part of pre-provasopressin (pre-proAVP). Pre-proAVP is a precursor protein which consists of a signal peptide, arginine vasopressin (AVP), neurophysin II and copeptin (1-3). These components are separated during axon transport from the cell body to the axon terminals in the posterior pituitary gland (3). On the path from the hypothalamus to the pituitary gland, copeptin and neurophysin II act as carrier proteins of AVP. Copeptin is stored in the neurohypophyseal vesicles together with AVP and neurophysin II until they are secreted (4). Schematic presentation of copeptin generation and maturation is shown in Figure 1. Copeptin also has a very important role in the proteolysis of pre-proAVP, but its main role in the circulation is still unknown (3,6). Slow degradation of copeptin in humans hints that this peptide may not have any significant function in the circulation (6). Copeptin is cosynthesized with AVP and is found in equimolar amounts with AVP in the circulation of healthy and critically ill subjects (7,8). Thus copeptin mirrors AVP concentration and can be used as a replacement biomarker of AVP release (6,7).
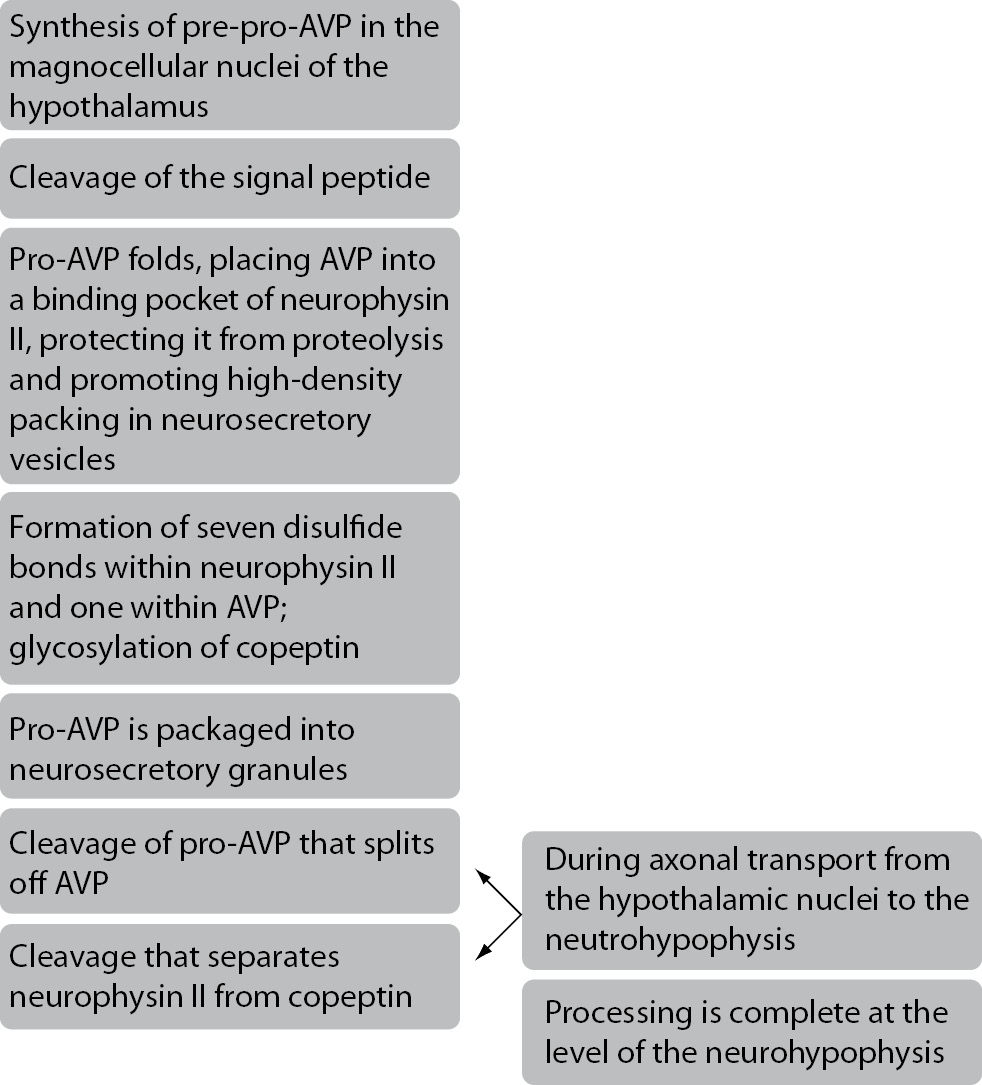
Figure 1. Schematic presentation of copeptin generation and maturation (5).
For certain diseases, diagnosis and prognosis can still be very demanding and time-consuming. This is why new biomarkers are needed to aid clinicians in making faster treatment decisions and more accurate prognostic assessment. To be of practical value, a new biomarker needs to provide quantitative information that is both reliable and reproducible. Moreover, the measurement of a biomarker should be easy, rapid and inexpensive to perform. Therefore, copeptin has been proposed as a prognostic marker in different illnesses and disorders where it may help in early detection and diagnostic accuracy. The purpose of this review is to summarize a handful of research done on copeptin and to discuss its role as a biological marker in the diagnosis and prognosis of various diseases such as acute myocardial infarction (AMI), heart failure, hyponatremia, sepsis, septic shock, infections of lower respiratory tract, acute dyspnea, vasodilatory shock, diabetes insipidus, autosomal dominant polycystic kidney disease (ADPKD), diabetes mellitus, metabolic syndrome, intracerebral hemorrhage, ischemic stroke and head injury.
Copeptin in circulation
Normal values of copeptin in healthy volunteers range between 1.70-11.25 pmol/L (6,9,10). Copeptin also shows minimal intra-individual variations (11). In healthy people, a 28h water deprivation leads to a twofold increase in concentration of copeptin, while infusion of hypotonic saline induces a 2-3-fold decrease of copeptin concentration (11). Copeptin concentration are lower in women when compared to men, but there is no significant difference in copeptin rise between men and womenwith changes in osmolality or volume status (3,12,13). Renal function also influences copeptin concentration in healthy men and this is not noticed in healthy women (12). Copeptin has not being found to correlate with age or estimated glomerular filtration rate in healthy subjects, except in patients with AMI where copeptin concentration correlates with age and estimated glomerular filtration rate (9,12,13). In advanced and acute heart failure the rise in copeptin concentration goes from 20 pmol/L to 45 pmol/L. In terms of life threatening conditions, such as severe sepsis, septic shock, hemorrhagic shock, ischemic stroke and AMI, copeptin increases to concentrations above 100 pmol/L. On the opposite sphere, copeptin decreases in patients with diabetes insipidus, hyponatremia and other conditions associated with reduced AVP concentration (13). It is still however unknown how copeptin is removed from the circulation. It is assumed that it is partially cleared through the kidneys because it is detectable in urine (7).
Function of AVP
AVP is a vasoactive neuropituitary hormone. It is one of the main hormones of the hypothalamic-pituitary-adrenal axis and its primary function is water regulation and homeostasis of electrolytes. The main stimulus for AVP release is hyperosmolarity. Hypotension, hypoxia, acidosis, infection, insulin-induced hypoglycemia, pain, nausea, vomiting, certain drugs and other non-specific causes of stress can also increase concentration of AVP in the circulation (3,7).
AVP binds to 3 different receptors, V1a, V1b and V2 receptor. They are classified into three subtypes based on their intracellular transduction mechanisms. The V1a and V1b receptors are associated with phosphoinositol turnover, while the V2 receptor activates adenylate cyclase (14). The V1a receptor is widely expressed and mediates AVPs prothrombotic and vasoconstrictor effects. Thus, AVP agonists are used in treating bleeding and hypotensive disorders (15-17). Through V1a receptor AVP also mediates liver glycogenolysis (16,17). The V1b receptor is expressed in the pituitary gland and pancreas (15,16). Through this receptor AVP stimulates the release of adrenocorticotropic hormone. Adrenocorticotropic hormone activates the hypothalamic-pituitary-adrenal axis and thus mediates a response to stress. Insulin and glucagon secretion are mediated via V1b receptor as well (16,17). The V2 receptor is expressed in the renal collecting ducts and through those receptors the antidiuretic effect of AVP is mediated (15,16). Pharmacological blockade of V2 receptor has been used to treat hyponatremia and heart failure (16,17).
Measurement of AVP and copeptin
Reliable measurement of plasma AVP concentration is difficult and is subject to preanalytical and analytical errors. Such difficulties stem from fact that AVP is unstable due to its short half-life of 24 min. To add to this problem, more than 90% of AVP in the circulation is bound to platelets, thus making accurate measurements very difficult (3,6,18,19). Incomplete removal of platelets from plasma samples or prolonged storage of unprocessed blood samples can lead to falsely elevated values of AVP. AVP is also unstable in isolated plasma and serum even when it is stored at -20 °C (6,13). The small size of AVP also challenges detection by sandwich immunoassays and therefore requires the use of less sensitive competitive immunoassays (13). All the above mentioned reasons contribute to the absence of routine AVP use in clinical practice (6).
Unlike AVP, copeptin is stable in EDTA plasma for up to 14 days at room temperature, while in citrate and heparin plasma copeptin is stable for 7 days (19). Its detection doesn’t require extraction or other complex preanalytical steps (13). It can easily be measured ex vivo by manual or fully automated chemiluminescence assays as a replacement biomarker for unstable AVP (1,20,21). Scheme of assay for the measurement of copeptin is shown in Figure 2. The assay requires minimal serum or plasma volume and the overall time for completing the analysis is between 20 and 30 minutes (13). Based on these facts, copeptin is suitable for routine measurements as an alternative to AVP (6). Some of the methodology characteristics for copeptin and AVP assays are summarized in Table 1.
Figure 2. Scheme of assay for the measurement of copeptin.
Table 1. Some of the methodology characteristics for copeptin and AVP assays (21,22).
Copeptin as a diagnostic and prognostic marker
In stressful situations such as illness, the relationship between plasma osmolality and AVP is lost because AVP with corticotropin-releasing hormone (CRH) leads to the production of adrenocorticotropic hormone (ACTH) and cortisol. Serum cortisol is proportional to stress levels, and by reflecting stress levels, cortisol predicts prognostic outcome in different diseases. However, cortisol is influenced by strong circadian rhythm and its measurement as a free hormone is demanding. These characteristics of cortisol place copeptin as a more reliable hormone for determination of stress levels. Of note, even mild to moderate stress situations contribute to the copeptin release. In recent years copeptin has being studied as a diagnostic and prognostic biomarker in various diseases (Table 2). Of mention are acute myocardial infarction, heart failure, acute exacerbation of chronic obstructive pulmonary disease, lower respiratory tract infections, acute dyspnea, sepsis, hemorrhagic and septic shock, diabetes mellitus, metabolic syndrome, hyponatremia, vasodilatory shock, diabetes insipidus, autosomal dominant polycystic kidney disease (ADPKD), intracerebral hemorrhage, ischemic stroke and traumatic brain injury (8,20). The data on diagnostic accuracy of copeptin in different disorders are shown in Table 3.
Table 2. Overview of the most important references on copeptin.
Table 3. Diagnostic accuracy of copeptin in different disorders.
Copeptin in acute myocardial infarction
Acute myocardial infarction (AMI) is a major cause of death in industrialized countries. Its prevalence in third-world countries is also steadily rising. Therefore, it is extremely important to ensure early detection and diagnostic accuracy. These abilities will aid rapid interventions in AMI and prevent premature discharge of unrecognized AMI patients from the emergency department (13). The most important methods in the diagnosis of AMI are electrocardiography (ECG) and determination of serum cardiac troponin T (cTnT) concentration, which complement clinical assessment. Cardiac Tn is superior to other biomarkers available for AMI diagnosis, including myoglobin, MB fraction of creatine kinase (CK-MB), myeloperoxidase and heart fatty acid-binding protein (23). Despite these methods and biomarkers, the exclusion of AMI is still demanding. ECG is of little help in the exclusion of AMI because one quarter to one third of patients with AMI show no significant ECG changes in the presence of ongoing acute cardiac ischemia. However, ECG is necessary for identification of patients with ST-segment elevation (24). Conventional cardiac biomarkers, such as CK-MB and TnT, are unable to aid in rapid exclusion of AMI in patients presenting early in the hospital with symptoms suggestive for AMI. This is due to the fact that their concentrations do not increase during the first few hours of AMI (25). Therefore, the exclusion of AMI requires monitoring of patients between a 6 to 9 hour period and serial blood sampling for measurement of cTnT concentration (24,26,27).
In order to increase the accuracy for detection of AMI, a highly sensitive cardiac troponin T (hs-cTnT) has been developed, which allows measurement of concentrations that are 10 times lower than the previous ones. This assay allows greater accuracy and earlier detection of AMI (28,29). However, despite its ability for earlier diagnosis of AMI, there is still a “troponin-blind” interval after the onset of chest pain still due to delayed troponin release (29). A use of this assay enables detection of myocardial injury due to a broad spectrum of pathologies which includes myocardial ischemic and myocyte physiologic remodeling. It increases the number of positive tests on admission as compared to the less sensitive assays which complicates triage and management of patients with chest pain (13).
If the organism is exposed to endogenous stress such as AMI, this activates the AVP system and copeptin is excreted into circulation independent of necrosis of cardiac cells (28). Also, inadequate filling of the left ventricle caused by AMI stimulates cardiac baroreceptors or causes direct damage to baroreceptors which subsequently leads to AVP and copeptin secretion from the posterior pituitary gland (13). After the onset of chest pain copeptin is elevated within 3-4 hours, reaching a peak value in the first day (26,29). During the second day they slowly start to decrease reaching a plateau after 3-5 days (29). From the pathophysiological aspect, decreasing concentration of copeptin just a day after myocardial ischemia may indicate adjustment to neurohumoral stress by activation of AVP system after AMI (29). A decrease in copeptin concentration may also be due to the cessation or at least a reduction of chest pain after the onset of AMI, or they may as well be an inter-play of both reasons (27). Copeptin rises at a time when other biomarkers are still undetectable (25,27). CK-MB, TnT and hs-cTnT concentrations reach peak values after more than 14 hours and return to their normal values slower (25). Copeptin is more sensitive than cTnT and myoglobin within the first 3 hours of AMI detection. The sensitivity of copeptin is 75.2% (cutoff > 9.8 pmol/L), cTnT 43% (cutoff > 0.03 μg/L) and myoglobin 62.3% (cutoff > 107 ng/mL) (30). However, in the final exclusion of AMI, copeptin is not able to replace cTnT, but only enables making earlier decisions in clinical practice (30). The combination of copeptin and cTn, compared with only cTn, improves diagnostic accuracy of AMI reaching a sensitivity of 85.1% and a negative predictive value (NPV) of 92.4% within 3 hours from the onset of chest pain. The highest sensitivity and NPV were achieved with 9.8 pmol/L as the cutoff representing the 95th percentile (30). Reichlin et al. reported even a greater sensitivity and NPV using both markers in exclusion of AMI. A copeptin concentration < 14 pmol/L in combination with a TnT ≤ 0.01 µg/L rules out AMI at presentation with a sensitivity of 98.8% and a NPV of 99.7% (27). Therefore, such combination of biomarkers would enable fast and accurate exclusion of AMI at an early stage of presentation without the need for serial blood sampling (24). This strategy of using more biomarkers (cTnT or hs-cTnT for detection and quantification of myocardial necrosis, and copeptin for quantification of endogenous stress) to assess the outcome after AMI has an advantage because it includes various pathological pathways and may be of prognostic significance (14,23,25, 27).
In a recent study Lotze et al. reported a positive correlation between hs-cTnT and copeptin at the time of initial AMI presentation (r = 0.41; P < 0.001). Cut-off value of 0.014 µg/L for hs-cTnT can be used for fast exclusion of AMI with a sensitivity of 92.3%, specificity of 53.5%, positive predictive value (PPV) of 16.7% and NPV of 98.6%. The corresponding values for copeptin are 69.2%, 47.3%, 11.7% and 93.9%, respectively. Cut-off value of 0.014 µg/L for hs-cTnT in combination with a copeptin value of < 14 pmol/L at admission exclude AMI with a sensitivity of 100%, specificity of 34.9%, PPV of 13.4% and NPV of 100%, indicating that this combination of biomarkers has a higher diagnostic accuracy than use of only hs-cTnT or copeptin. By measuring hs-cTnT with copeptin, AMI could be ruled out with a NPV of 100% in one third of patients who have symptoms which indicate to AMI (29). In addition, Meune et al. reported that the combination of hs-cTnT and copeptin at admission compared with use of only hs-cTnT increased detection of acute coronary syndrome (ACS) on the level which is similar to serial measurement of hs-cTnT. The combination of hs-cTnT and copeptin at admission excludes ACS with a sensitivity of 86.7% and NPV of 82.6% (hs-cTnT value of 0.014 μg/L is the 99th percentile of the assay; cut-off value of copeptin is 14 pmol/L). The sensitivity of only hs-cTnT measured at admission was 73.3% and NPV was 76.5%, while sensitivity and NPV of hs-cTnT measured after 3 hours were 83.3% and 83.9%, respectively (28).
Copeptin concentration is significantly higher in patients with suspected ACS with final diagnosis of AMI compared to patients with suspected ACS with a different final diagnosis. These diagnoses include unstable angina and other cardiac and non-cardiac causes of chest pain (25). Patients with unstable angina have similar copeptin concentration like patients with other causes of chest pain (27). Therefore, ischemia, as long as not accompanied by necrosis (e.g. unstable angina), does not cause higher secretion of copeptin than other causes of chest pain. Also, copeptin cannot discriminate patients with unstable angina from patients with non ischemic chest pain (27).
Copeptin and heart failure
Currently there are several neurohormones which are used as biomarkers for diagnosis, prognosis and clinical decision in chronic heart failure. The B-type natriuretic peptide (BNP) and N-terminal proBNP (NT-proBNP) are the most common. They are released in the circulation when cardiac stretching occurs and they are powerful prognostic biomarkers of heart failure outcome (19,31). But despite these biomarkers, it is considered that heart failure is not detected in up to 50% of patients, so diagnosis of this disease remains a major challenge (31). Therefore, scientific research has lately been directed towards finding new biomarkers that can improve the diagnosis and prognosis of heart failure.
It is noticed that AVP concentration is increased in patients with chronic heart failure (16,19,31). Since AVP secretion is partially stimulated in response to a high osmolality, there are still unanswered questions as to why we find an increase of AVP in patients with heart failure who generally have a lower osmolality (16,31). There are several possible explanations for this increase. The release of AVP in heart failure is mainly stimulated by reduced cardiac output, and in turn insufficient filling of arteries leads to carotid sinus and aortic arch baroreceptor activation. It seems that in heart failure, AVP secretion caused by osmolarity stimulus is overridden by the non-osmolarity stimulus, which leads to hypo-osmolality. Some studies showed that hyponatremia, which is often present in patients with heart failure, might also stimulate the release of AVP. It is also known that angiotensin II increases concentration of AVP. In heart failure angiotensin II is elevated even in the presence of ACE inhibitors (16,19).
AVP serves as a parameter of disease severity and it appears to have a role in the pathology of heart failure as well. AVP by stimulation of V1a receptors increases protein synthesis in myocytes, it stimulates the development of myocardial hypertrophy and decreases heart contractility and also stimulates cardiac fibroblasts, and thus promotes myocardial fibrosis. Increased release of AVP is also associated with increased preload, increased filling pressures and increased afterload (31). High copeptin concentration which reflects rise of AVP in the blood therefore predicts poor prognosis in patients with chronic heart failure (16,18). Copeptin is a good predictor of death or heart failure within 60 days, helping to classify patients into groups of low, medium and high risk. It is also independent of already established conventional risk factors for heart failure (16,20). Copeptin is not only important for short-term prognosis but is also important in identifying patients with long-term risk. Increased copeptin concentration in elderly patients with symptoms of heart failure is associated with increased risk of mortality after a follow-up of 13 years (20).
Copeptin reflects different pathological pathways that lead to a deterioration of heart failure than BNP or NT-proBNP, so when copeptin is combined with BNP or NT-proBNP, predicting disease outcome can be improved (19). Some studies indicate the equal prognostic value of copeptin, BNP and NT-proBNP in predicting mortality, whereas some studies give a better prognostic value to copeptin (14,16,19,32,33). A possible explanation for better prognostic value of copeptin is that BNP and NT-proBNP, unlike copeptin, correlate with age and renal function. Also there is large variation in BNP in plasma over time in chronic heart failure. However, the combined measurement of plasma copeptin and NT-proBNP certainly provides more valuable information than the measurement of only one biomarker (14,16).
Neuhold et al. investigated the predictive ability of copeptin for the entire spectrum of heart failure, including patients with New York Heart Association (NYHA) functional class I to IV. They reported that copeptin at all symptomatic stages of heart failure was independently associated with mortality, and it was the most powerful predictor of death in II and III NYHA class. However, it is noteworthy that for asymptomatic patients (NYHA functional class I) rate of events was too low to ensure information in this cohort. For patients in the most severe stage of heart failure (NYHA functional class IV) copeptin has provided additional independent information, but was inferior to the values of sodium and glomerular filtration (33).
Copeptin and hyponatremia
Distinguishing patients with syndrome of inappropriate antidiuretic hormone secretion (SIADH) from patients with hyponatremia in clinical practice is a diagnostic challenge. Measuring copeptin concentration is not reliable enough to differentiate these two conditions due to large overlaps in values of copeptin, even though mean values of copeptin are higher in patients with hyponatremia compared to patients with SIADH (34). However, it has been proven that the ratio of copeptin in serum and sodium in urine is diagnostically very useful (34). This ratio can be used in differentiating normovolemic hyponatremia (ratio ≤ 30 pmol/mmol) as SIADH, from hypovolemic hyponatremia (ratio > 30 pmol/mmol), i.e. in differentiation of inappropriate from appropriate secretion of AVP (15,34).
Higher copeptin concentration is seen in patients with hyponatremia during chronic renal failure compared to patients with gastrointestinal loss. This is probably due to several reasons, such as activation of the AVP system in patients with impaired renal function, abundance of receptors for AVP in renal tubules, and the fact that copeptin is excreted from the body via the kidneys (35). Measuring copeptin concentration in plasma of patients with acute illnesses seems to provide little information about sodium imbalance because copeptin concentration is similar in different etiologies of sodium imbalance. It is worth mentioning that though copeptin concentration may be similar in different etiologies, it may still contribute to understanding the pathophysiology of various causes of hyponatremia (35).
Copeptin, sepsis and septic shock
AVP and copeptin are also elevated in sepsis and septic shock (4,6,36). Jochberger et al. reported that AVP concentration is higher in patients with sepsis compared to patients with infections without systemic inflammation. AVP secretion can be induced by inflammatory mediators such as interleukin 1, tumor necrosis factor α and several other factors such as acidosis, pain, hypoxia or neuroendocrine stress. These latter factors are also likely to occur in patients with severe sepsis than in patients with infections without systemic inflammation (36). In this study, AVP values did not differ between patients with severe sepsis with shock and those without shock, or between surviving patients and those who didn’t survive. In septic shock higher values of AVP are expected because of reduced venous filling as well as arterial hypotension which stimulate the release of AVP. However, during severe sepsis and primarily during septic shock, AVP system is partially dysfunctional and relative AVP deficiency contributes to the inability to establish vascular tone in patients with septic shock. Proposed mechanisms for possible sepsis associated dysfunction of AVP system include autonomic dysfunction of afferent pathways and inadequate production of AVP with consequent depletion of storage in the neuro-hypophysis. In patients with severe sepsis AVP in plasma is associated with serum osmolarity, arterial pH, oxygen partial pressure and arterial lactate, whereas in patients with septic shock AVP is associated only with serum osmolarity (36).
In sepsis and septic shock significantly higher plasma copeptin has been noticed as well. Although there is an excellent correlation between copeptin and AVP in different diseases, their correlation in severe sepsis and septic shock is smaller. The need for continuous veno-venous hemofiltration (CVVHF) worsens the correlation between copeptin and AVP, suggesting that different sieving coefficients may result in diverse amounts of filtered hormone levels during CVVHF. Inflammation can also interfere with the relationship between AVP and copeptin (36). Unlike the AVP whose values don’t differ in patients with sepsis who survived and those who didn’t survive, copeptin is higher in non-survivors compared to survivors (15,36,37). This suggests that copeptin could represent a prognostic biomarker in sepsis (15,37).
Copeptin and infections of lower respiratory tract
In infections of lower respiratory tract, gas exchange in the lungs causes changes in AVP system (38). Endotoxins and acute phase cytokines (e.g. IL-1β, IL-6, TNF-α) which are present during respiratory infections also stimulate the secretion of AVP (39). However, AVP also has an important role in regulation of the hypothalamic-pituitary-adrenal axis through which it mediates its response to stress in diseases (39). Copeptin is cosynthesized with AVP, it is found in equimolar amounts with AVP in the circulation, and therefore copeptin concentration is significantly higher than in healthy individuals. Copeptin concentration was measured in patients with community-acquired pneumonia (CAP), ventilator associated pneumonia (VAP), acute bronchitis and chronic obstructive pulmonary disease (COPD). It was found that in those diseases copeptin concentration increased with the severity of the disease (15,39-42). It has been shown that copeptin is an independent predictor of mortality in VAP (15,40-42). Due to the complexity of AVP effects in VAP, the difference in copeptin concentration according to survival status is small, and therefore cannot be defined with a clear cutoff value. Biomarker that predicts the outcome of a disease should be elevated in that disease compared to healthy individuals, and it must reflect the degree of organ dysfunction and overall health condition. Boeck et al. reported that copeptin fulfills the first two criteria, but lacks considerably in the third criterion. Therefore, its predictive power is lost in the heterogeneous picture of VAP (38).
In CAP copeptin is the only biomarker that is an independent predictor of this disease, and may provide additional information about the severity of disease. Due to its high NPV, copeptin may help in identifying patients with low risk of death. Since it is independent of patient’s age, it can be a valuable prognostic factor in young patients with CAP (43). Despite its very good prognostic value, copeptin gives little information about predicting the etiology of CAP because there is considerable overlap in copeptin values between patients with different etiologies of pneumonia (43). Krüger et al. reported that it is clinically important to consider antibiotic therapy for correct interpretation of copeptin concentration as well as concentration of other inflammatory biomarkers in CAP, because patients treated with antibiotics showed significantly lower copeptin values compared to those who didn’t take antibiotics before admission to the hospital, which can be explained by reduced circulation damage caused by sepsis (40).
In severe chronic obstructive pulmonary disease (COPD) AVP has a vasoconstrictive effect. At insufficient tissue oxygenation this response makes cardiovascular adaptation easier through elevated AVP concentration. Elevated AVP compensates for insufficient activation of V1 receptors after exposure to ongoing hypoxemia. It is also considered that in pulmonary hypertension AVP creates a negative inotropic effect on the right ventricle and increases pulmonary vasoconstrictive response in endotoxemia. This could link increased AVP and copeptin with poor clinical outcome in COPD (40). Compared with CRP and procalcitonin, copeptin is superior in predicting the course of COPD exacerbation. Elevated copeptin concentration predicts the final outcome in patients with acute exacerbation of COPD and serves as a risk factor for long-term clinical failure, regardless of age, comorbidity, hypoxemia or functional pulmonary disorder (44).
Müller et al. compared copeptin values in various etiologies of lower respiratory tract infections and reported that copeptin concentration in patients with acute bronchitis and acute exacerbation of COPD was significantly higher compared to control group. On further examination it was also discovered that there was no difference in copeptin concentration between acute bronchitis and acute exacerbation of COPD. Also, patients who were diagnosed with CAP had the highest copeptin concentration. Only slightly elevated copeptin concentration in acute bronchitis and acute exacerbation of COPD compared to control group probably reflects localized infection (39).
Copeptin and acute dyspnea
The identification of dyspneic patients at the highest risk for adverse outcomes is difficult because acute dyspnea often occurs due to several reasons such as cardiac, pulmonary or inflammatory causes (45). In these patients copeptin secretion is probably connected with three possible mechanisms. Firstly, AVP released in heart failure is mainly driven by inadequate filling of arteries which activates the carotid sinus and aortic arch baroreceptors. Secondly, in severe COPD AVP has vasoconstrictive effect which is induced by hypoxia. Elevated concentration of AVP can compensate for the inadequate regulation of V1 receptors after exposure to ongoing hypoxemia. Finally, copeptin is significantly elevated in bacterial infection and febrile conditions (45).
Potocki et al. pointed out that copeptin concentration is significantly higher in patients with acute decompensated heart failure (ADHF) compared to patients with other diagnoses responsible for acute dyspnea. Also, in that study, copeptin was significantly higher in patients who did not survive compared to patients who survived at 30 days, regardless of the presence of ADHF. Therefore, copeptin could be a promising prognostic biomarker for short term mortality in patients with acute dyspnea and even more in patients with ADHF. Copeptin is also independent of natriuretic peptides values (45).
Copeptin and vasodilatory shock
Copeptin concentration in plasma is elevated in patients with severe vasodilatory shock. These elevated copeptin reflects the severity of the disease, a higher incidence of complications and a greater risk of death. AVP is infused in patients with severe vasodilatation with the intention of supplementing a lack of endogenous AVP and reducing the use of potentially high and toxic doses of catecholamine. During therapy with exogenous AVP, copeptin concentration is decreased, suggesting the suppression of endogenous AVP system. High copeptin before treatment and the extent of decrease of copeptin during treatment with exogenous AVP is associated with severity of disease and mortality (46).
Copeptin and diabetes insipidus
AVP is released into the circulation after hypoglycemic stimulus. Insulin-induced hypoglycemia is used as a standardized procedure to assess the integrity of the hypothalamic-pituitary-adrenal axis. In patients with normal pituitary function, insulin-induced hypoglycemia leads to a threefold increase of AVP concentration. Since copeptin strongly correlates with AVP, copeptin can separate healthy people from patients with diabetes insipidus. Copeptin concentration measured 45 minutes after injection of insulin has the best sensitivity and specificity for detection of diabetes insipidus. Copeptin has good, but not perfect discriminatory capacity (2).
Copeptin and kidneys disease
Copeptin is associated with autosomal dominant polycystic kidney disease (ADPKD) as well. ADPKD is the most common hereditary kidney disease which is characterized by progressive cyst formation in both kidneys. AVP binds to V2 receptors in the distal tubules and collecting ducts promoting water absorption and production of cAMP. cAMP plays an important role in the stimulation of cyst growth in the kidney. Since copeptin reflects AVP concentration, measurement of copeptin can provide valuable information about the severity of ADPKD. Higher copeptin concentration is associated with poor renal function, lower effective renal blood flow, larger kidneys and albuminuria. Copeptin is associated with plasma osmolarity, but not with 24-hour urine volume, 24-hour urine osmolarity or fractional excretion of urea. In people with lower glomerular filtration, the fact that copeptin is partly cleared by the kidney probably isn’t an explanation for higher concentration of copeptin because increased copeptin concentration would induce a lowering of plasma osmolarity, which would lead to a decrease of copeptin (47).
Copeptin, diabetes mellitus and metabolic syndrome
Activation of the hypothalamic-pituitary-adrenal axis by AVP in chronic psychosocial stress is one of the mediators associated with insulin resistance, diabetes mellitus, obesity and metabolic syndrome (16,48). AVP directly stimulates the secretion of cortisol by activating V1a receptors, which interferes with insulin activity, stimulates glucagon secretion and glycogenolysis. This process subsequently leads to an increase in blood glucose. AVP also by activating the V1b receptors on the chromaffin cells in the adrenal medulla increases epinephrine which also contributes to the development of hyperglycemia through glycogenolysis in the liver (48). Raised AVP, as a result of resistance of AVP to V1a receptor, might also contribute to insulin resistance and development of diabetes mellitus by stimulation of V1b receptor (16). Therefore, copeptin could be a new potential biomarker of insulin resistance and diabetes mellitus.
Copeptin is also associated with several components of metabolic syndrome including obesity, dyslipidemia, elevated concentration of triglycerides and low HDL-cholesterol. The association of copeptin with higher triglyceride concentration may be secondary to increased hepatic triglyceride synthesis due to glucocorticoids, glucagon and epinephrine released under stress (all of which are regulated by AVP) (48). Enhörning et al. reported that elevated copeptin concentration at baseline predicted the incidence of diabetes mellitus and abdominal obesity during a long-term follow-up of 15.8 years on average. The association between copeptin at baseline and the incidence of diabetes mellitus is independent of the incidence of abdominal obesity and vice versa, so it is possible that AVP independently triggers two different pathways leading to diabetes mellitus and abdominal obesity. Despite this, there is the possibility that a primary elevation of AVP by increasing abdominal fat deposition leads to the development of diabetes mellitus (49).
Copeptin and central nervous system diseases
In intracerebral hemorrhage (ICH) formation of edema in the brain indicates an unfavorable outcome. It has been shown that blockade of AVP receptors reduces brain edema. This suggests that AVP has an important role in the formation of brain edema (17,50,51). Thus, measurement of copeptin can indicate existing or developing brain edema which can help in identifying patients with increased risk of edema formation (50). Also, after ICH copeptin concentration in plasma is significantly increased, reflecting the severity of hemorrhagic damage and predicting long-term clinical outcome. Copeptin also helps to identify patients with risk of early neurological deterioration and correlates with hematoma volume, which is directly associated with clinical severity and outcome after ICH (50-52). Other biomarkers (D-dimer, glutamate, matrix metalloproteinases and protein S100b) can also predict early neurological deterioration and mortality in patients with ICH by way of different pathophysiological processes. Therefore, it is advised that prognostic assessments and decisions about management of ICH rely on few parameters where copeptin as a new prognostic biomarker is used in combination with other biomarkers (50). Copeptin, unlike the other brain biomarkers, is directly secreted into the systemic circulation. This fact gives relevance to copeptin measurement in ICH (17). In other conditions, such as ischemic stroke, aneurysmal subarachnoid hemorrhage and head injury, copeptin concentration is elevated as well (53). In this group of patients high copeptin concentration in peripheral blood is associated with mortality and poor neurologic outcome (51). Yu et al. reported that copeptin increases with severity of brain injury and therefore, its measurements after brain injury provides an opportunity to distinguish patients with a one-year good or poor outcome (17).
Conclusion
Copeptin, as a biomarker released into the circulation under endogenous stress, could be a potentially promising biomarker in diagnosis of various diseases and prediction of functional outcomes. Since it is not specific to a certain disease, copeptin could be used as an adjunct with more specific biomarkers where it may increase diagnostic accuracy and aid clinicians in making better diagnostic judgements. However, copeptin is still in its infancy and more research is needed in order to prove its clinical usefulness.