Introduction
Anti-Müllerian hormone (AMH) is a 140-kDa dimeric glycoprotein hormone and belongs to the transforming growth factor-β (TGF-β) family. AMH is synthesized as a large precursor with a short signal sequence followed by the pre-prohormone that forms homodimers. Prior to secretion, the mature hormone undergoes glycosylation and dimerization to produce a 144-kDa dimer composed of identical disulphide-linked 72-kDa monomer subunits. Each monomer contains an N-terminal domain (also called the “pro region”) and a C-terminal domain (also called the “mature region”); it is believed that N-terminal domain accentuates the activity of the C-terminal domain in which resides the bioactivity of the molecule (1). During cytoplasmic transit, between 5 and 20% of AMH is cleaved at a specific site between the N-terminal and the C-terminal domain of the 72-kDa monomer, to form two polypeptides of 58-kDa (pro region) and 12-kDa (mature region). These two parts of the molecule remain in noncovalent attachment. The human gene coding for AMH is located on the short arm of chromosome 19; it has been sequenced and isolated (2).
AMH signaling pathway
Target organs for AMH in males are Müllerian ducts and, in both sexes, gonads. The structure of the specific receptor for AMH has been isolated and characterized (3). As a member of the TGFβ family of growth factors, it is thought that AMH uses the signal transduction system that has been identified for the other factors of the family, notably TGFβ itself, activin and the bone morphogenetic proteins (BMPs). These factors signal through a serine–threonine kinase receptor complex consisting of ligand-specific type II receptors and more general type I receptors, also known as activin receptor-like protein kinases (ALKs). An activated receptor complex phosphorylates and activates cytoplasmic Smad proteins that translocate to the nucleus and directly or indirectly affect gene expression (Figure 1). For AMH one type II receptor has been identified (AMHRII) and shown to be specific and necessary for AMH signaling (4). The gene coding for AMH receptor is located on the long arm of chromosome 12 (5). The AMHRII gene is specifically expressed in the gonads and in the mesenchymal cells adjacent to the Müllerian ducts. Besides the exclusive AMHRII, three candidate AMH type I receptors have been identified to be involved in AMH-induced Müllerian duct regression. However, the relative contribution of these three type I receptors to AMH signaling in the ovary remains to be determined (6).
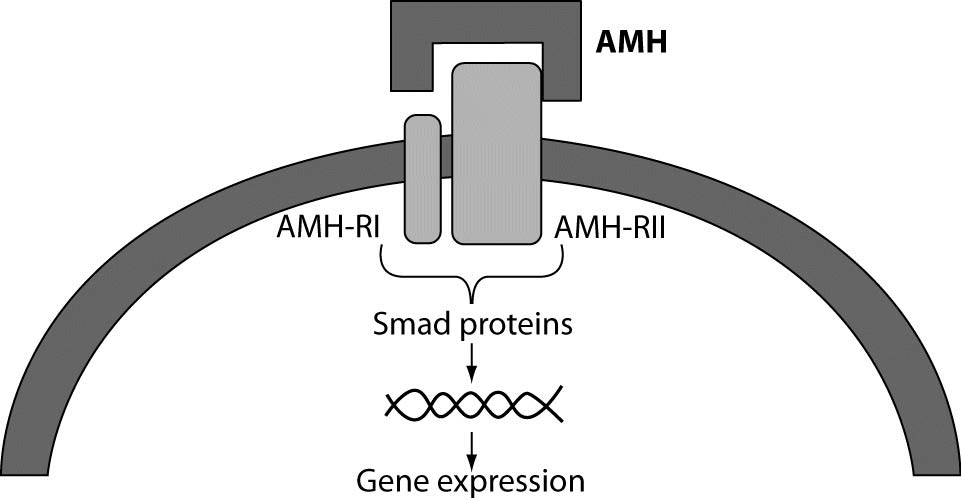
Figure 1. AMH receptor binding. The primary receptor, type II, binds its ligand and is co-expressed with type I receptor which serve as a signal transducer. After being activated, the type I receptor is phosphorylated by AMH-RII. The activated type I receptor, in turn, phosphorylates downstream Smads proteins. Phosphorylated receptor-specific Smads translocate to the nucleus where they regulate gene expression (60).
AMH throughout the human life
Prenatal stage
AMH plays fundamental role in fetal sex differentiation. Before the 7th week of gestation, male and female fetuses have indifferent gonads, bipotential external genitalia, and two pairs of unipotential internal ducts (the Müllerian ducts and the Wolffian ducts). In the XY fetus, the expression of the SRY gene triggers testicular differentiation (7). From the 8th gestational week, the somatic cells of the developing testes, the Leydig cells and Sertoli cells, produce three hormones that are essential for the correct differentiation of the individual in the male direction. The steroid hormone testosterone, produced by the Leydig cells, stimulates development of the male sex characteristics, such as differentiation of the Wolffian ducts into epididymides, vasa deferentia and seminal vesicles (7). Insulin-like factor 3, a member of the relaxin–insulin family, is also produced by the Leydig cells, and is an essential signal for the first phase of testes descent. AMH is produced by the Sertoli cells of the testes and is responsible for the regression of the Müllerian ducts that in the female fetus differentiate into the oviducts, the uterus and the upper portion of the vagina (8,9). Absent during female sex differentiation, AMH is first expressed after 36 weeks of intrauterine life in the granulosa cells of ovaries (10).
From birth to puberty
In prepubertal boys, Leydig cells produce low amounts of testosterone. Sertoli cells are still immature and spermiogenesis is arrested in a premeiotic stage, while AMH remains secreted at high level till the onset of puberty (9). Therefore, serum AMH in male infants reflects the function of testes reliably and can also be used in cryptorchidic males as the initial laboratory test to assess the presence of the testes (9).
In newborn girls, ovaries contain primordial follicle pool containing 1-2 million of oocytes arrested in the diplotene stage of meiotic prophase I and surrounded by flattened pre-granulosa cells (11). These primordial follicles stay in their arrested state for years till the onset of puberty. AMH is expressed in granulosa cells of growing follicles and continues to be present throughout the reproductive ages (4). In girls, AMH circulating values are almost undetectable at birth with a slight increase within the first years of age, prior to puberty.
Puberty and adulthood
In boys, with the onset of puberty the secretion of hypothalamic gonadotropin releasing hormone (GnRH) and both gonadotropins, luteinizing hormone (LH) and follicle-stimulating hormone (FSH), increase. Leydig cells undergo further differentiation. Testosterone synthesis increases, which also invokes maturation of Sertoli cells. Germinal cells undergo meiosis and spermatogenesis begins. The inhibitory effect of testosterone prevails over FSH stimulation, resulting in down-regulation of AMH expression and the circulating levels rapidly decrease. The secretion of AMH reaches values in adult males and is maintained almost constant until the rest of men’s life (9).
In girls, the rise of ovarian estradiol synthesis and activation of the hypothalamus–pituitary–ovarian axis occurs with the onset of puberty. Oocytes at the first meiotic prophase undergo atresia or resume meiosis after activation by an ovulation-inducing LH surge to form the haploid gamete for fertilization (11). AMH is produced by ovaries and secreted into the bloodstream. It is first expressed in granulosa cells of the recruited primordial follicles and continues to be expressed in the growing follicles in the ovaries until they have reached the size of about 4-6 mm. At that differentiation state, usually one follicle is selected for dominance and its further growth is controlled by action of pituitary FSH (12). Possible actions of AMH in the ovary are presented in figure 2.
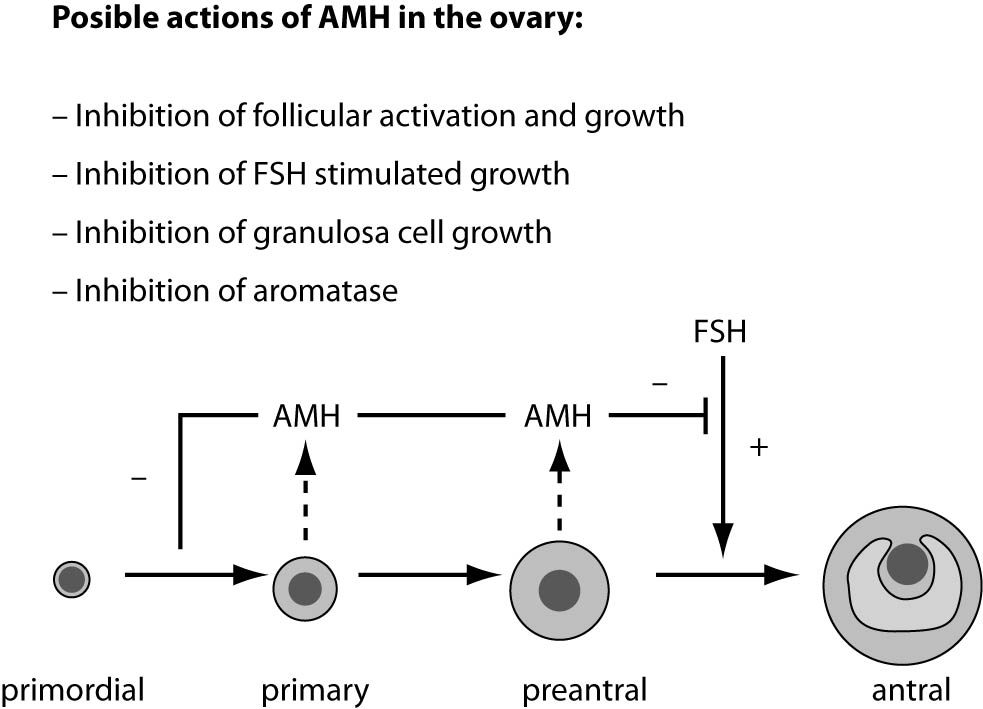
Figure 2. Model of AMH actions in the ovary. AMH is expressed in primary, pre-antral and small antral follicles. Initial recruitment of primordial follicles takes place as a continuous process, whereas cyclic recruitment is driven by a rise in FSH serum levels at the end of a previous menstrual cycle. AMH inhibits (–) the initial recruitment of primary follicles from the resting primordial follicle pool and the stimulatory effect of FSH on the growth of preantral and small antral follicles (+) (4).
Physiology and pathophysiology of AMH in males
Anti-Müllerian hormone is the earliest Sertoli cell hormone secreted in males and, together with inhibin B and FSH, is an important indicator of Sertoli cell function (13). The postnatal proliferation of Sertoli cells is essential for spermatogenesis, during which the transient GnRH surge occurs. In the first weeks of newborn male, the infantile GnRH surge induce a significant increase of gonadotropins (LH and FSH), followed by the increased testosterone production in Leydig cells, as well as AMH and inhibin B in Sertoli cells of testes (9). These unexpected high levels of androgens and AMH in the first months after birth are explained by the physiological androgen insensitivity of Sertoli cells in these periods due to the lack of androgen receptor expression in Sertoli cell nuclei (9).
This stage, so called „mini-puberty“, has been proposed as the first opportunity to detect patients with hypogonadotropic hypogonadism (HH), since it cannot be observed in boys with congenital HH (14). Thus, decreased AMH serum level, compared to that in infants of the corresponding age, is an early biochemical marker of congenital central hypogonadism. AMH levels remain high during the whole prepubertal phase and are downregulated in puberty by increased testosterone levels. Serum AMH levels in males, according to age, are shown in table 1 (15).
Table 1. Serum AMH in normal males.
The assessment of AMH (and inhibin B) can be useful in the differential diagnosis of constitutional delay of growth and puberty when AMH serum levels remain at high prepubertal values, while low AMH (and inhibin B) levels at the age of physiological puberty are indicative for the congenital hypogonadism (9).
Klinefelter syndrome is the most common congenital cause of hypergonadotropic hypogonadism. The main clinical features include gynecomastia, small testes, absent spermatogenesis, normal or reduced Leydig cell function and elevated FSH levels. This disorder is caused by a supernumerary X chromosome (karyotype 47,XXY or mosaicism 46,XY/47,XXY). At the time when normal puberty is expected, laboratory findings are characterized by the significantly increased FSH and LH levels, low testosterone, decreased AMH and undetectable inhibin B serum levels (17).
Assessment of AMH (and inhibin B) represents a promissory approach in other causes of male hypogonadism. For example, acquired hypergonadotropic hypogonadism can be caused by autoimmune diseases, chemotherapy and/or radiation therapy for malignant neoplasm that affect testicular tissue and function (18).
The measurement of circulating AMH can also be useful in infants with bilateral non-palpable gonad (cryptorchidism) as evidence that the testes can be present but not descended. If necessary, the hCG stimulation test is recommended to assess the presence of functioning testicular tissue and the detection of defects in testosterone biosynthesis by Leydig cells and its action (18).
AMH seems to be a good candidate marker for incomplete or reduced spermatogenesis since it is specifically secreted by Sertoli cells in both serum and seminal fluid in detectable concentrations. Serum AMH seems to be significantly lower in non-obstructive azoospermic men than in those with obstructive azoospermia and normal fertile man. However, the wide overlapping of values between subjects prevents this hormone from being of clinical utility (19).
If Sertoli cells are unable to produce AMH, because of mutations in the AMH gene, or if target organs are unresponsive to its action through mutations of the AMHRII gene, Müllerian duct derivatives will be retained in genetic male, otherwise normally masculinized. This condition is known as the persistent Müllerian duct syndrome (PMDS). In patients with mutations of the AMH gene, levels of circulating AMH are extremely low, even before puberty, when AMH levels are normally high. AMH concentrations are higher in patients with receptor type II (AMHRII) gene mutations (20).
The role of AMH in ovarian reserve evaluation
AMH is produced by granulosa cells of growing follicles, from pre-antral to antral stage, until they reach the size of 4-6 mm (21). AMH expression disappears in follicle of increasing size and is almost lost in follicles larger than 8 mm (22). The main physiological role of AMH in the ovary seems to be targeted to the inhibition of primordial follicle recruitment, thus preventing too early depletion of the follicular reserve. Both in vivo and in vitro experiments have indicated that the transition from primordial into growing follicles becomes enhanced in absence of AMH (23). Furthermore, the inhibition of sensitivity to FSH by AMH is a decisive step in follicular selection and it may occur due to the variable expression of AMH receptors among the recruited small pre-antral follicles. In such circumstances, out of the initially recruited follicle units, only those with lower AMH expression become sensitive to FSH, of which usually one is permitted for dominance. Therefore, AMH acts as an autocrine factor that regulates dominant follicle selection. Current theories also suggest a role for AMH as a co-regulator of steroidogenesis in granulosa cells, as AMH levels appear to be related to estradiol levels in follicular fluid from small antral follicles (24). This is confirmed in a study which showed that polymorphisms in the gene for AMH or AMHRII seem to be related to follicular phase estradiol levels, suggesting a role for AMH in the FSH-induced steroidogenesis in the human ovary (25).
The expression of AMH in pre-antral and small antral follicles mainly contributes to its serum levels during the reproductive life. An active cohort of growing follicles represents the antral follicle count (AFC), which also serves as a marker of ovarian reserve and strongly correlates with serum AMH levels (26). Despite this correlation, AFC poorly reflect the pre-antral follicle pool and, moreover, requires state-of-the-art ultrasound machine and experienced ultrasonographist (27). Therefore, AMH may provide a more accurate assessment of the oocyte/follicle pool. Contrary to the other ovarian reserve markers, AMH can be measured at any time during the menstrual cycle due to insignificant intra-cycle variability, which is a great advantage in clinical practice (28,29). In the longitudinal study of serum AMH levels in white, black and Hispanic women, authors found certain, but insignificant differences in measured values in relation to race/ethnicity (30). AMH levels are not changed during pregnancy, during which endogenous gonadotropin release is substantially diminished (31). In addition, AMH levels remain stable during oral contraceptive administration (32). These findings are consistent with the concept that AMH levels reflect the continuous FSH-independent non-cyclic growth of small follicles in the ovary. Hence, AMH is a unique endocrine parameter for the investigation of the ovarian reserve (33).
Still, the Freeman’s cross-sectional study in healthy, regularly menstruating women has shown a significant inverse association of AMH serum levels and body-mass index (BMI) (34). In obese women, mean AMH levels were 65% lower than AMH levels of non-obese women. In addition, a multivariable regression analysis of independent association of variables BMI, age, menopausal status and race with AMH confirmed that BMI remained significantly associated with AMH levels. The authors suggested two possible explanations for the finding: either obesity may be associated with decreased ovarian reserve, or obesity is associated with follicular dysfunction. The weakness of the study is that blood samples were selected in a cohort of women in late reproductive ages (35-47 years) and the distribution of studied variables may not be representative for the entire range of reproductive life.
AMH as indicator of ovarian aging throughout reproductive life
Trends in modern societies have been accomplished also with maternity postponement and the increasing demand for assisted reproduction techniques (ART) (35). Because of a considerable proportion of subfertile women, the assessment of ovarian reserve becomes of crucial interest in women presenting to fertility clinics.
As a woman ages there is a gradual decrease in both quantity and quality of the oocytes residing within follicles, accompanied by a concomitant decrease in AMH serum concentration. It has been demonstrated that AMH levels correlate strongly with AFC and are more reliable in predicting oocyte supply than woman’s age and other conventional serum markers (FSH, estradiol) (36). Investigation of age-dependent reference values for AMH should provide a better insight in cutoff levels throughout the reproductive life of women. Recently, in two extensive studies, age-specific distributions of AMH in female population were examined (37,38). In women, between 25 and 35 years of age, the average decrease in the median serum AMH value is 1.4 pmol/L (0.2 ng/mL) per year, while after age of 35, the average yearly decrease is 0.7 pmol/L (0.1 ng/mL). The rate of decline in mean AMH values is 1.4 pmol/L (0.2 ng/mL) per year through age 40 and diminishes to 0.7 pmol/L (0.1 ng/mL) per year thereafter (37).
During the years before the final menstrual period, oocytes undergo an accelerated rate of loss until their pool is mostly depleted. A hypothesis stands that this process is initiated when the total of number of oocytes reaches approximately 25,000 and that woman, under physiological condition, reaches this threshold at about 37-38 years of age (42). At menopause, the number of oocytes has decreased to some hundreds (43).
Perhaps, one of the outstanding challenges nowadays is the lack of reference values of AMH in healthy female population of reproductive age (39). This is mostly due to the fact that the data have been obtained from infertility clinics and/or centers for reproductive medicine where the majority of women undergo laboratory tests for ART procedures. Shebl and coworkers presented basal serum AMH levels in women who attended an ART program because of male factor (38). This presumably healthy cohort consisted of ovulatory women in reproductive ages, without issues related to abnormal ovarian function (e.g., tubal factor, endometriosis, polycystic ovaries). Evaluated median concentrations and confidence intervals for basal serum AMH according to age are shown in table 2 (supplementary data available
here).
Another two studies from Scotland have been published most recently, proposing a population model for serum AMH levels (40,41). According to the observations, AMH values exhibit the greatest heterogeneity and spread in absolute values in younger women, suggesting that they are presenting with a wider range of development of AMH-expressing follicular cohorts as compared with older women. Also, it may be that they exhibit differential AMH expression for their age matched follicular pool (40). The model of the association of AMH concentrations with age confirmed a nonlinear decreasing rate of AMH with age; the nomogram for women between 25 and 45 years is best described by a quadratic equation: log AMH = a + b x Age + c x Age2. In this equation, log AMH is natural logarithm of AMH, a = 1.932 (95% confidence interval – CI, 1.891 to 1.973), b = -0.138 (-0.149 to -0.127) and c = -0.003 (-0.005 to -0.002) (40). In the subsequent study, Kelsey and coworkers generated the first validated model of serum AMH in healthy females from conception to menopause; the results have been derived from 3,260 data extracted from previously published control and/or prospective studies in healthy female subjects (41). They have even included the data from cord blood of preterm infants up to those in females of 54.3 years of age. As to the model of serum AMH through the females’ life, concentrations fall shortly after birth, increase slightly at about two years of age and then rise significantly with the onset of puberty. Interestingly, the authors suggest that AMH serum levels reach a peak at about 24.5 years of age and then gradually decrease, in a nonlinear manner, through the period of women’s reproductive life (41).
For years, serum levels of FSH and estradiol have been the decisive biochemical markers in assessment of diminishing ovarian function, but FSH tends to rise over basal values in follicular phase of the menstrual cycle only when ovarian reserve is significantly exhausted and ovarian function already compromised (44). More favorable results have been obtained using AMH as a marker, for it reflects the follicular cohort as a whole, including still inactive follicles and those being transited from primordial to the early antral stage. It has been assumed that a threshold of 2.8 pmol/L (0.39 ng/mL) for AMH has the optimal combined sensitivity and specificity for prediction of the onset of menopause in six years, with a positive predictive value of 0.90 (95% CI, 0.81-0.96) and negative predictive value of 0.76 (95% CI, 0.65-0.86), respectively (45).
AMH as a marker of premature ovarian senescence
It is considered that follicular exhaustion becomes obvious in the fourth decade of woman’s life when changes in the gonadal environment occur, although the majority of women of that age continue with apparently normal menstrual cycles (46). The most recent study in a population of presumably healthy women has shown that in approximately 5% of women of all ages, AMH serum levels are ≤ 7 pmol/L (1 ng/mL) (38). This finding has undoubtedly pointed to the fact that all age subgroups comprise women with a reduced ovarian reserve and that even young women are at risk of decreased reproductive potential.
Premature ovarian failure is clinically defined as the complete absence of menstrual cycles before the age of 40, with FSH levels exceeding 40 IU/L and AMH levels below the menopausal threshold or even undetectable in most of the cases (46). Premature ovarian ageing represents a milder degree of gonadal dysfunction which is characterized with baseline FSH < 12 IU/L and/or abnormally low age-specific AMH (46,47). It has been argued that AMH offers better clinical specificity for the reason that FSH is mostly reflective of late-stage, gonadotropin-sensitive, follicles preceding ovulation (48). In another words, AMH and FSH differently reflect ovarian reserve at different stages of life.
The prestigious results in the recent Gleicher’s studies postulate that the increasing triple CGG (Cytosine-Guanine-Guanine) repeats on the FMR1 (fragile X) gene correlate with specific ovarian reserve parameters, and are associated with premature ovarian senescence and infertility in young women (49). The significant associations between FMR1 genotypes and AMH levels could allow predictions about the functional ovarian reserve already in young ages (50).
AMH as a predictor of success in assisted reproduction techniques (ART)
The reproducibility of AMH between consecutive cycles in the same woman, in distinction from FSH, estradiol and inhibin B, permits clinicians to have a reliable serum marker in prediction of ovarian response in assisted reproduction cycles. Much data show a strong and positive correlation between basal AMH serum levels and the number of retrieved oocytes in women undergoing ovarian stimulation (35). Moreover, basal serum AMH value and antral follicle count (AFC) have shown a similar power in prediction of the number of retrieved oocytes (35,51). In meta-analysis of about 30 studies, receiver operating characteristic (ROC) curves for the prediction of ovarian response indicated no significant difference between the performances of AMH and AFC (52). The number of developed follicles and the number of retrieved oocytes are two of the most important criteria for defining poor response in women undergoing ovarian stimulation for in-vitro fertilization (IVF) (35).
For instance, Barad and co-workers found that AMH cut-off value of 3.5 pmol/L (0.5 ng/mL) could predict the retrieval of less than four oocytes with a specificity of 84% and sensitivity of 87% (48). At this discriminatory AMH value, the positive and negative predictive values were 79.4% and 90%, respectively. In contrast, a value of FSH > 12 IU/L had sensitivity of 64.5% and specificity of 82.2%, with positive and negative predictive values of 71.4% and 77%, respectively (51).
Ultimately important is, however, whether assessment of AMH can predict chance for pregnancy and live births in IVF cycles. In the next study of the mentioned authors, maximal ROC inflections, which differentiate between better and poorer livebirth chances in women with diminished ovarian reserve (DOR), were at AMH cut-off < 7.5 pmol/L (1.05 ng/mL). Still, it was demonstrated that clinical pregnancies could be established at all AMH level, even in the absence of detectable AMH (53).
AMH as a marker of ovarian pathophysiology
Polycystic ovarian syndrome (PCOS)
PCOS is the most frequent endocrine disorder of ovarian function, with a prevalence of 6-7% among women of reproductive age (54). It has been known for years that it represents more complex entity than solely an ovarian dysfunction. Increased androgen synthesis, disrupted folliculogenesis and insulin resistance are the manifestation of endocrine/reproductive and metabolic disturbances of the impaired molecular mechanisms known to underlie PCOS. According to the Rotterdam consensus, the diagnosis should meet at least two, of the three following criteria: chronic anovulation or oligomenorrhea, clinical or biochemical hyperandrogenism and polycystic ovarian morphology (55). An ultrasound examination reveals increased ovarian volume and/or the presence of ≥ 12 follicles in each ovary. It has been hypothesized that the increased intrafollicular AMH levels, found in PCOS, lower the follicle sensitivity to circulating FSH, thus preventing follicle selection and resulting in follicle arrest at the small antral phase (2-5 mm) (56). The balance between FSH and AMH might be crucial for aromatase activity at the time and after the selection of the dominant follicle. Decreased FSH action inhibits aromatase activity in synergy with AMH, hindering the conversion of androgens to estrogens, thus contributing to ovarian androgen excess and reduction of follicular production of estradiol. The resulting low levels of estradiol may also contribute to the failure of follicle selection. Increased AMH levels may also act directly on theca cells to stimulate androgen synthesis (54).
Circulating AMH levels in women with PCOS are two to three times higher than in healthy controls, corresponding to the increase in the number of small follicles in ovaries (54).
As a diagnostic marker of PCOS, AMH has been found to offer a relatively high specificity and sensitivity (92% and 67%, respectively) (56). Moreover, evaluation of basal AMH levels in women with PCOS plays an important role in predicting the risk for ovarian hyper-response and informing a clinician to direct the application of mild stimulation protocols in order to avoid ovarian hyperstimulation syndrome (OHSS) (35,36).
Polymorphisms of AMH and AMHRII genes as causes of infertility
Two genes are responsible for the AMH signal transduction pathway (AMH gene and its receptor AMHRII gene), confirming their association with follicular phase estradiol level (25). AMH is produced by granulosa cells in early developing follicles, which indicates its role during the initiation of primordial follicle growth and in regulation of FSH sensitivity. As shown in a recent study, genetic variants of AMH and AMHRIIgenes seem to be associated with idiopathic infertility in normo-estrogenic and normo-ovulatory women (57). The results suggest that AMHpolymorphisms could modify hormone biological activities, playing a role in controlling follicle recruitment and growth. Although in the small sample size, the genotyping has shown that allele frequencies of –482 A>G, IVS 5–6 C>T, IVS 10+77 A>G, 146T>G polymorphisms are statistically significantly increased in the infertile women compared with the controls (57). Although it seems plausible that polymorphisms of AMH and AMHRII might reduce the hormone biologic activity, making follicles more sensitive to FSH, further investigations are expected to clarify their association with unexplained infertility in women.
Müllerian aplasia or Mayer-Rokitansky-Küster-Hauser syndrome
Müllerian agenesis, also named the Mayer-Rokitansky-Küster-Hauser syndrome (MRKHS), is the second most common cause of primary amenorrhea (58). Although the etiology of the syndrome remains unclear, evidences suggest that MRKHS is transmitted in an autosomal dominant manner causing defects in prenatal development in the female genital tract. It is characterized by the congenital absence of the Müllerian structures including the Fallopian tubes, the uterus, and the internal portion of the vagina in an otherwise chromosomally normal 46,XX subject. Females with MRKHS have functioning ovaries and normal external genitalia. Still, molecular analyses of AMH and AMHRIIgenes have not detected any deleterious mutations responsible for the syndrome (59). This finding reflects normally regulated AMH gene promoters, transcription and AMH protein production in adult MRKH patients. As blood AMH levels decrease over time and are associated with the number of remaining antral follicles, AMH therefore represents a specific marker for ovarian age and could be helpful for predicting successful IVF or surrogacy pregnancies in such cases (58).
AMH as a marker of granulosa cell tumors
The sex cord stromal neoplasms are derived from mesenchymal stem cells in the ovarian cortex; they include granulosa–theca cell tumors and granulosa cell tumors (GCTs), which constitute 1-3% of all ovarian tumors (60,61). Two distinct types of GCTs have been described on the basis of clinical presentation and histological characteristics: the juvenile and the adult form (60). GCTs are hormone-secreting neoplasms; they may produce estradiol and progesterone and they secrete peptide hormones, such as inhibin and AMH (61). Therefore, serum AMH serves as biochemical marker in diagnosis and monitoring of the disease. However, serum AMH is elevated only in sex cord stromal tumors, while inhibin may be elevated in different types of cancer (60). Globally, AMH serum levels seem to be elevated in 76-93% of GCT patients (61). Although AMH is considered a powerful inhibitor in granulosa cell proliferation, studies have shown that the inhibitory signal is functional only in the initial stage of tumor development, but not when the tumor becomes progressive and reach a large dimension (60). One of the possible theories is that, in progressive tumors, the ability of binding of AMHRII receptor by its ligand is lost and that the inhibitory signal of AMH becomes inefficient (61).
Unfortunately, the agents used to treat the malignancies are able to destroy a greater or lesser number of ovarian primordial follicles in cancer survivors (62). Radiotherapy and chemotherapy, especially alkylating agents, cyclophosphamide, bisulphan, melphalan, cytosine, procarbazine and cis-platin are extremely harmful to the ovarian reserve and function (63). In such cases, there is a definite and unquantifiable benefit of monitoring serum AMH levels, because girls and young women are at increased risk of premature ovarian failure as well as of reduced reproductive ability. Bath and coworkers have shown that, among patients with regular menstrual cycles, cancer surviving women have significantly lower basal AMH values than controls (13.0 ± 3.0 vs. 21.0 ± 3.4 pmol/L; P < 0.05) and higher early follicular phase FSH levels (7.5 ± 1.4 vs. 4.2 ± 0.3 IU/L; P = 0.02) (63). In another study, Partrige and coworkers investigated parameters of ovarian reserve in women treated with tamoxifen and radiotherapy for early stage breast cancer (64). In univariate logistic analyses, AFC, FSH, AMH and inhibin B levels were all significantly different between survivors and controls, though in a multiple logistic regression analysis, AMH levels distinguished survivors from controls with the greatest statistical difference (odds ratio 7.63) (64). Although the both studied groups, patients and controls, were adequately matched, the limitation of the study is that the parameters of ovarian reserve had not been measured before chemotherapy started.
Development of immunoassays for AMH measurement
The first reported AMH immunoassay used a pair of monoclonal antibodies raised against human recombinant AMH, both directed to epitopes in the pro region (65). However, the use of this assay showed results with variability in AMH concentrations due to storage and freeze-thaw instability. The second type of assay used a pair of monoclonal antibodies, one directed to the pro region and the other to the mature region of the AMH molecule (61). A third assay, developed more recently, uses a monoclonal antibody pair directed to epitopes in the pro region (66). The new AMH Gen II assay uses a pair of monoclonal antibodies directed to epitopes in the mature region of AMH (67). The advantage of this assay is that measured values are not affected by proteolysis of AMH in the sample as the mature region is more stable against proteolysis compared to the pro region, in part due to its multiple cystine residues (67). In addition, the Gen II assay measures AMH in human, monkey, bovine, other mammalian species with improved performance. The lowest amount of AMH in a sample that can be detected with 95% probability is 0.57 pmol/L (0.08 ng/mL). The monoclonal antibodies are developed against the epitopes of the mature region of AMH which prevents any of cross reactions with inhibin A, LH and FSH at 2 times their physiological concentration. In addition, potential interfering substances from serum matrix (hemoglobin, triglycerides and bilirubin) do not deteriorate acceptable limits of ± 10% difference to the value of control specimen (68).
AMH Gen II assay, developed by Beckman Coulter Company (Beckman Coulter Inc., California, USA), uses antibodies developed by Diagnostic Systems Laboratories (DSL Inc., Texas, USA) and has been standardized to the Immunotech calibration (Immunotech a.s., Check Republic) (67). The lowest amount of AMH in a sample that can be detected with 95% probability is 0.56 pmol/L (0.08 ng/mL). Passing-Bablok regression analysis has shown a good correlation with the Immunotech AMH ELISA (r = 0.99; P < 0.001; y = 1.0x) (67). Yet, it has to be taken into account that laboratories implementing Beckman Coulter AMH Gen II assay should expect an increase in AMH values of approximately 40% (69).
As it is a relatively new assay, a need exists for inter-laboratory comparison in the form of an external quality assurance scheme (70). Even more, laboratories that have implemented AMH immunoassay in a routine practice should enroll in the external proficiency testing program. The latest has recently been established under UK NEQAS scheme design (71).
Conclusion
Extensive investigation of clinical significance of AMH serum values undoubtedly confirms that it is not just a “hand of biologic watch”. One may think that the scientists have researched all the conditions when AMH serves as a biochemical marker of male and female physiology and patophysiology. Certainly, we should be aware of an outstanding role of AMH in different stages of human life. Perhaps more prospective long term studies in healthy population are needed to evaluate the reliable reference values according to ages, especially in women within the reproductive life span. This could facilitate a correct evaluation of ovarian reserve in infertile subjects, allow a more accurate prediction of reproductive potential and improve counseling in particular cases.
Notes
Potential conflict of interest
None declared.
References
1. Wilson C, Clemente N, Ehrenfels C, Pepinsky R, Josso N, Vigier B, et al. Mullerian inhibiting substance requires its N-terminal domain for maintenance of biological activity, a novel finding within the transforming growth-factor-beta superfamily. Mol Endocrinol 1993;7:247-57.
2. Picard JY, Benaroust R, Guerrier D, Josso N, Khan A. Cloning and expression of cDNA for anti-Mullerian hormone. Proc Natl Acad Sci 1986;83:5464-8.
3. di Clemente N, Wilson C, Faure E, Boussin L, Carmillo P, Tizard R, et.al. Cloning, expression, and alternative splicing of the receptor for anti-Mullerian hormone. Mol Endocrinol 1994;8:1006–20.
4. Visser JA, Themmen APN. Anti-Müllerian hormone and folliculogenesis. Mol Cell Endocrinol 2005;234:81-6.
5. La Marca A, Volpe A. Anti-Müllerian hormone (AMH) in female reproduction: is measurement of circulating AMH a useful tool? Clin Endocrinol 2006;64:603-10.
6. Visser JA. AMH signalling from receptor to target gene. Mol Cell Endocrinol 2003;211:65-73.
7. Rhoades RA, Bell DR, eds. Medical physiology: principles for clinical medicine. 3rd ed. Baltimore, Philadelphia; Lippincott Wiliams&Wilkins; 2009. p. 716-9.
8. Lee MM, Donahoe PK. Müllerian-inhibiting substance: a gonadal hormone with multiple functions. Endocr Rev 1993;14:152-64.
9. Grinspon RP, Rey RA. Anti-Müllerian hormone and Sertoli cell function in pediatric male hypogonadism. Horm Res Paediatr 2010;73:81-92.
10. Rajpert-De Meyts E, Jorgensen N, Graem N, Muller J, Cate RL. Skakkebaek NE. Expression of anti-Müllerian hormone during normal and pathological gonadal development: association with differentiation of Sertoli and granulosa cells. J Clin Endocrinol Metab 1999;84:3836–44.
11. Speroff L, Glass RH, Kase NG in Clinical Gynecologic Endocrinology and Infertility. Mitchell C (ed.), Williams & Wilkins, Baltimore, Philadelphia, Hong Kong, Munich, Sidney, Tokyo, 1994, p. 362-6.
12. Alviggi C, Humaidan P, Howles CM, Tredway D, Hillier SG. Biological versus chronological ovarian age: implications for assisted reproductive technology. Available at: http://www.rbej.com/content/7/1/10, Accessed September 22, 2009.
13. Aksglaede L, Sørensen K, Boas M, Mouritsen A, Hagen CP, Jensen RB, et al. Changes in Anti-Müllerian Hormone (AMH) throughout the Life Span: A Population-Based Study of 1027 Healthy Males from Birth (Cord Blood) to the Age of 69 Years. J Clin Endocrinol Metab 2010;95:5357-64.
14. Brito VN, Berger K, Bilharinho Mendonca B. Male hypogonadism: childhood diagnosis and future therapies. Pediatric Health 2010;4:539-55.
15. Rey AR, Belville C, Nihoul-Fékété C, Michel-Calemard L, Forest MG, Lahlou N. Evaluation of Gonadal Function in 107 Intersex Patients by Means of Serum Antimüllerian Hormone Measurement. J Clin Endocrinol Metab 1999;84:627-31.
16. Marshall WA, TannerJM. Variations in pattern of pubertal changes in girls. Arch Dis Child 1969;44:291–303.
17. Wikström AM, Dunkel L. Testicular function in Klinefelter syndrome. Horm Res 2008;69:317–26.
18. Viswanathan V, Eugster EA. Etiology and treatment of hypogonadism in adolescents. Endocrinol Metab Clin N Am 2009;38:719–38.
19. Tüttelmann F, Dykstra N, Themmen AP, Visser JA, Nieschlag E, Simoni M. Anti-Müllerian hormone in men with normal and reduced sperm concentration and men with maldescended testes. Fertil Steril 2009;91:1812–9.
20. Belville C, Josso N, Picard JY. Persistence of Müllerian derivatives in males. Am J Med Genet 1999;89:218-23.
21. La Marca A, Broekmans FJ, Volpe A, Fauser BC, Macklon NS. Anti-Müllerian hormone (AMH): what do we still need to know? Hum Reprod 2009;24:2264-75.
23. Nilsson E, Rogers N, Skinner MK. Actions of anti-Mullerian hormone on the ovarian transcriptome to inhibit primordial to primary follicle transition. Reproduction 2007;134:209–21.
24. Andersen CY, Byskov AG. Estradiol and regulation of anti-Müllerian hormone, inhibin-A, and inhibin-B secretion: analysis of small antral and preovulatory human follicles’ fluid. J Clin Endocrinol Metab 2006;91:4064–9.
25. Kevenaar ME, Themmen AP, Laven JS, Sonntag B, Fong SL, Uitterlinden AG, et al. Anti-Müllerian hormone and anti-Müllerian hormone type II receptor polymorphisms are associated with follicular phase estradiol levels in normo-ovulatory women. Hum Reprod 2007;22:1547–54.
26. van Disseldorp J, Lambalk CB, Kwee J, Looman CWN, Eijkemans MJC, Fauser BC, Broekmans FJ. Comparison of inter- and intra-cycle variability of anti-Müllerian hormone and antral follicle counts. Hum Reprod 2010;25:221-7.
27. Knauff EAH, Eijkemans MJC, Lambalk CB, ten Kate-Booij MJ, Hoek A, Beerendonk CCM. Anti-Müllerian hormone, inhibin B, and antral follicle count in young women with ovarian failure. J Clin Endocrinol Metab 2009;94:786-92.
28. La Marca A, Stabile G, Carducci Artenisio A, Volpe A. Serum anti-Müllerian hormone throughout the human menstrual cycle. Hum Reprod 2006;21:3103-7.
29. Tsepelidis S, Devreker F, Demeestere L, Flahaut A, Gerrvy C, Engelrt Y. Stable serum levels of anti-Müllerian hormone during the menstrual cycle: a prospective study in normo-ovulatory women. Hum Reprod 2007;22:1837-40.
30. Seifer DB, Golub ET, Lambert-Messerlian G, Benning L, Anastos K, Watts D, et al. Variations in serum Müllerian inhibiting substance between white, black and Hispanic women. Fertil Steril 2009;92:1674-8.
31. La Marca A, Giulini S, Orvieto R, De Leo V, Volpe A. Anti-Müllerian hormone concentrations in maternal serum during pregnancy. Hum Reprod 2005;20:1569-72.
32. Streuli I, Fraisse T, Chapron C, Bijaoui G, Bischof P, de Ziegler D. Serum antimüllerian hormone levels remain stable throughout the menstrual cycle and after oral or vaginal administration of synthetic sex steroids. Fertil Steril 2008;90:395-400.
33. Steiner AZ. Clinical implications of ovarian reserve testing. Obstet Gynecol Survey 2009;64:120-8.
34. Freeman EW, Gracia CR, Sammel MD, Lin H, Chong-Leong Lim L, Strauss III JF. Association of anti-mullerian hormone levels withobesity in late reproductive-age women. Fertil Steril 2007;87:101-6.
35. La Marca A, Sighinolfi G, Radi D, Argento C, Baraldi E, Carducci Artenisio A, et al. Anti-Müllerian hormone (AMH) as a predictive marker in assisted reproductive technology (ART). Hum Reprod Update 2009;16:113-30.
36. Nardo LG, Gelbaya TA, Wilkinson H, Roberts SA, Yates A, Pemberton P, et al. Circulating basal anti-Müllerian hormone levels as predictor of ovarian response in women undergoing ovarian stimulation for in vitro fertilization. Fertil Steril 2009;92:1586-93.
37. Seifer DB, Baker VL, Leader B. Age-specific serum anti-Müllerian hormone values for 17,120 women presenting to fertility centers within the United States. Fertil Steril 2011;95:747-50.
38. Shebl O, Elmer T, Sir A, Schreirer-Lechner E, Mayer RB, Tews G, et al. Age-related distribution of basal serum AMH level in women of reproductive age and a presumably healthy cohort. Fertil Steril 2011;95:832-4.
39. Guidi GC, Salvagno GL. Reference intervals as a tool for total quality management. Biochem Med 2010;20:165-72.
40. Nelson SM, Messow MC, Wallace AM, Fleming R, McConnachie A. Nomogram for the decline in serum antimüllerian hormone: a population study of 9,601 infertility patients. Fertil Steril 2011;95:736-41.
41. Kelsey TW, Wright P, Nelson SM, Anderson RA, Wallace WHB. A validated model of serum anti-Müllerian hormone from conception to menopause. PLoS ONE 2011;6:e22024.
42. Sowers MFR, Eyvazzadeh AD, McConnell D, Yosef M, Jannausch ML, Zhang D, et al. Anti-Müllerian hormone and inhibin B in the definition of ovarian aging and the menopause transition. J Clin Endocrinol Metab 2008;93:3478-83.
43. Faddy MJ, Gosden RG. A model conforming the decline in follicle numbers to the age of menopause in women. Hum Reprod 1996;11:1484-6.
44. van Montfrans JM, Hoek A, van Hooff MH, de Koning CH, Tonch N, Lambalk CB. Predictive value of basal follicle-stimulating hormone concentrations in a general subfertility population. Fertil Steril 2000;74:97-103.
45. Tehrani FR, Solaymani-Dodaran M, Azizi F. A single test of antimüllerian hormone in late reproductive-aged women is a good predictor of menopause. Menopause 2009;16:797-802.
46. de Carvalho BR, Rosa e Silva AC, Rosa e Silva JC, dos Reis RM, Ferriani RA, Silva de Sá MF. Ovarian reserve evaluation: state of the art. J Assist Reprod Genet 2008;25:311-22.
47. Knauff EAH, Eijkemans MJC, Lambalk CB, ten Kate-Booij MJ, Hoek A, Beerendonk CCM. Anti-Müllerian hormone, inhibin B, and antral follicle count in young women with ovarian failure. J Clin Endocrinol Metab 2009;94:786-92.
48. Barad DH, Weghofer A, Gleicher N. Comparing anti-Müllerian hormone (AMH) and follicle-stimulating hormone (FSH) as predictors of ovarian function. Fertil Steril 2009;91:1553-5.
49. Gleicher N, Weghofer A, Barad DH. Discordances between follicle stimulating hormone (FSH) and anti-Müllerian hormone (AMH) in female infertility. Available at: http://www.rbej.com/content/8/1/64, Accessed June 17, 2010.
50. Gleicher N, Weghofer A, Barad DH. Defining ovarian reserve to better understand ovarian aging. Available at:http://www.rbej.com/content/9/1/23, Accessed February 7, 2011.
51. Jayaprakasan K, Campbell B, Hopkisson J, Johnson I, Raine-Fenning N. A prospective, comparative analysis of anti-Müllerian hormone, inhibin-B, and three-dimensional ultrasound determinants of ovarian reserve in the prediction of poor response to controlled ovarian stimulation. Fertil Steril 2010;93:855-64.
52. Broer SL, Mol BW, Hendriks D, Broekmans FJ. The role of anti-Mullerian hormone in prediction of outcome after IVF: comparison with the antral follicle count. Fertil Steril 2009;91:705-14.
53. Gleicher N, Weghofer A, Barad DH. Anti-Müllerian (AMH) defines, independent of age, low versus good livebirth chances in women with severely diminished ovarian reserve. Fertil Steril 2010;94:2824-7.
54. Diamanti-Kandarakis E. Polycystic ovarian syndrome: pathophysiology, molecular aspects and clinical implications. Expert Rev Mol Med 2008;10;e3.
55. The Rotterdam ESHRE ASRM-sponsored PCOS Consensus Workshop Group: Revised 2003 consensus on diagnostic criteria and long-term health risks related to polycystic ovary syndrome. Fertil Steril 2004;81:19-25.
56. Pigny P, Jonard J, Robert Y, Dewailly D. Serum anti-Müllerian hormone as a surrogate for antral follicle count for definition of the polycystic ovary syndrome. J Clin Endocrinol Metab 2006;91:941-5.
57. Rigon C, Andrisani A, Forzan M, D’Antona D, Bruson A, Cosmi E, et al. Association study of AMH and AMHRII polymorphisms with unexplained infertility. Fertil Steril 2010;34:1244-8.
58. Oppelt P, Strissel PL, Kellermann A, Seeber S, Humeny A, Beckmann MW, Strick R. DNA sequence variations of the entire anti-Müllerian hormone (AMH) gene promoter and AMH protein expression in patients with the Mayer–Rokitansky–Küster–Hauser syndrome. Human Reproduction 2005;20:149–57.
59. Zenteno JC, Carranza-Lira S, Kofman-Alfaro S. Molecular analysis of the anti-Müllerian hormone receptor. and galactose-1-phosphate uridyl transferase genes in patients with the Mayer-Rokitansky-Küster-Hauser syndrome. Arch Gynecol Obstet 2004;269:270-3.
60. La Marca A, Volpe A. The anti-Müllerian hormone and ovarian cancer. Hum Reprod Update 2007;13:265-73.
61. Long WQ, Ranchin V, Pautier P, Belville C, Denizot P, Cailla H, et.al. Detection of minimal levels of anti-Müllerian hormone during follow-up of patients with ovarian granulosa-cell tumor by means of a highly sensitive enzyme-linked immunosorbent assay. J Clin Endocrinol Metab 2000; 85:540-4.
62. Wallace WHB, Barr RD. Fertility preservation for girls and young women with cancer: what are remaining challenges? Hum Reprod Update 2010;16:614-6.
63. Bath LE, Wallace WHB, Shaw MP, Fitzpatrick C, Anderson RA. Depletion of ovarian reserve in young women after treatment for cancer in childhood: detection by anti-Müllerian hormone, inhibin B and ovarian ultrasound. Hum Reprod 2003;18:2368-74.
64. Partrige AH, Ruddy KJ, Gelber S, Schapira L, Abusief M, Meyer M, et al. Ovarian reserve in women who remain premenopausal after chemotherapy for early stage breast cancer. Fertil Steril 2010;94:638-44.
65. Hudson P, Douglas I, Donahoe P, Cate R, Epstein J, Pepinsky RB, MacLaughlin D. An immunoassay to detect human Mullerian inhibiting substance in males and females during normal development. J Clin Endocrinol Metab 1990;70:16-22.
66. Al-Qahtani A, Muttukrishna S, Appasamy M, Johns J, Cranfield M, Visser JA, et al. Development of a sensitive enzyme immunoassay for anti-Mullerian hormone (AMH) and the evaluation of potential clinical applications in males and females. Clin. Endocrinol (Oxf) 2005;63:267-73.
67. Kumar A, Kalra B, Patel A, McDavid L, Roudebush WE. Development of a second generation anti-Müllerian hormone (AMH) ELISA. J Immunol Methods 2010;362:51-9.
68. Dodig S. Interferences in quantitative immunochemical methods. Biochem Med 2009; 19:50-62.
69. Wallace AM, Faye SA, Fleming R, Nelson SR. A multicentre evaluation of the new Beckman Coulter anti-Müllerian hormone immunoassay (AMH Gen II). Ann Clin Biochem 2011;48:370-3.
70. Sciacovelli L, Secchiero S, Zardo L, Plebani M. The role of the External Quality Assessment. Biochem Med 2010;20:160-4.